
By confining single ions of aluminium and magnesium in an electric trap, cooling them to near absolute zero and probing them with laser beams, physicists at the National Institute of Standards and Technology (NIST) in Boulder, Colorado have built what is in effect the world’s most accurate clock. Having fractionally improved on the performance of another clock at NIST, the researchers have shown that their device would neither gain nor lose a second in 33 billion years (if it could run for that long). Such accurate timekeeping, they say, could boost geodesy and lead to new insights in fundamental physics.
The clocks that currently underpin atomic time rely on precisely measuring the frequency of microwaves emitted during a specific transition in caesium atoms. But such devices are limited by the relatively low frequency of that radiation. To keep time even more accurately, and eventually introduce a new definition of the second, physicists are developing clocks based on higher-frequency optical transitions.
The latest work at NIST features what is known as a quantum-logic clock. Built by Samuel Brewer and colleagues, it uses a positive ion of aluminium-27 as its timekeeper. When exposed to ultraviolet laser light at wavelength 267 nm, the ion undergoes a transition with a very narrow linewidth – making its frequency very well defined. What is more, that transition is largely immune to sources of external noise – such as blackbody radiation – that in other types of optical clock shift the frequency away from its true value.
A magnesium-25 ion is used to cool the aluminium down to the very low temperatures needed to minimize thermal noise. Cooling involves the absorption of photons at another specific frequency, but practical limitations mean that this cannot be done using the aluminium itself. This is because the required frequency in is too high for any practical laser. By entangling the two ions, the magnesium cools the aluminium via Coulomb interactions. This process also allows the quantum state of the aluminium ion to be read-out following exposure to the clock laser.
Achilles’ heel
In their research, Brewer and team have been addressing the clock’s remaining Achilles’ heel – the fact that aluminium has a relatively low mass. Held in a trap using oscillating electric fields, the aluminium can move around the trap more easily than a heavier ion. This creates a problem of relativistic time dilation, a slight shift in the ion’s transition frequency due to its finite speed. An uncertainty of one part in 1018 corresponds to a mere 40 cm/s – “a slow walking speed”, as Brewer puts it. “That has dominated the [clock’s] uncertainty for the last ten years or so,” he says.
The researchers had to deal with two distinct sources of motion. One occurs at the frequency of the trap’s oscillating fields (about 40 MHz) due to a residual field causing the ion to move away from the centre of the trap. By making the trap slightly more symmetrical and reducing the drive frequency, Brewer says that the team has lowered the uncertainty in this source of motion by a factor of ten.
They also reduced time dilation due to “secular motion”, which is related to the temperature of the ions in the trap. Brewer explains that the trap functions as a 3D harmonic oscillator, and with a finite temperature the ion occupies a distribution of motional states. The idea, he says, is to try and put the ion in its ground state in all three dimensions. By getting close to this using what is known as “pulsed Raman sideband cooling”, he and his colleagues were able to reduce the uncertainty in the secular motion by about a factor of 15.
Ytterbium pipped at the post
After fine-tuning some other aspects of the clock mechanism, the researchers totted up all the estimated systematic uncertainties. Their tally came to 9.4×10−19, which just pips the 1.4×10−18 achieved last year by Andrew Ludlow and colleagues, also at NIST, with an optical clock made from a “lattice” containing thousands of ytterbium atoms.
In so doing, both groups have taken the first step along a roadmap that was drawn up by a group of metrologists at the International Committee for Weights and Measures last year. Outlining what needs to be done before the second can be redefined in terms of an optical frequency, the roadmap says that at least three different clocks must demonstrate a systematic uncertainty “about two orders of magnitude” lower than the best caesium devices (which achieve about 1.6×10-16).
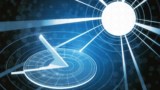
A brief history of timekeeping
With optical clocks evolving quickly, Brewer says it is not yet clear which technology – and hence transition – will be chosen to redefine the second. He points out that lattice clocks tend to be more stable and so can reach their stated accuracies more quickly. But he believes that the aluminium-ion clock is “as good a candidate as any other” being developed. He and his colleagues are currently working on a new version of their clock with a further improved trap and vacuum, which, he says, might reduce systematic uncertainty by a further factor of ten.
As well as bringing in a new definition of the second, Brewer says that optical clocks might also improve geodesy by exploiting the difference in ticking rate that gravitational redshift induces at different altitudes. In fundamental physics, meanwhile, ultra-accurate clocks could be used to search for any variation in the fine structure constant. That, in turn, he says, might even lead to new searches for extremely light dark matter –which could modify the value of the fine structure constant.
The ion clock will be described in an upcoming paper in Physical Review Letters
- NIST team member David Hume talks about the aluminium ion clock in this episode of the Physics World Weekly podcast.