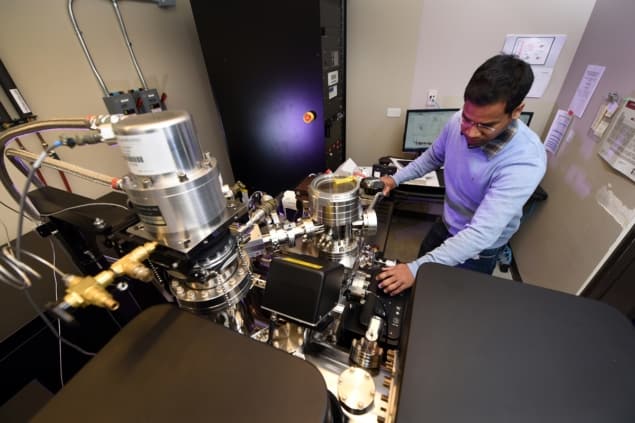
Developing materials capable of withstanding high-temperature environments has been a major concern for materials scientists in recent years due to their demand in aerospace engineering and solar energy applications. These ‘superalloys’ contain multiple components and are often of complex structure. Researchers at the Idaho National Laboratory have studied the hierarchical structure of a novel superalloy on both the nanoscale and microscale to understand its origins and its role in enhancing the material stability at high temperatures.
Superalloys is the name given to a group of metallic alloys that are especially stable at high temperatures (> 800 °C) and they are usually based on iron, titanium, cobalt, or nickel. The defining characteristic of these materials is the presence of two distinct phases, referred to as γ and γ’. Typically these phases exist in a form where precipitates of the γ’ phase are embedded in within the larger γ phase. It is these γ’ precipitates that undergo “coarsening”, a process where small grains grow at the expense of larger ones to form new morphologies, at elevated temperatures.
Coarsening ultimately leads to degraded mechanical properties and shorter lifetimes. In this case a team of researchers led by Subhashish Meher have analysed the chemical composition and physical morphology of a hierarchically structured γ-γ’ superalloy system in order to understand the mechanisms through which the phase hierarchy forms and leads to enhanced resistance against coarsening.
The higher stability from within
The material developed by Meher and colleagues is similar to the conventional γ-γ’ structure, however in this case the γ’ precipitates also contain even smaller precipitates of the γ, hence they use the term “hierarchy”. The researchers used transmission electron microscopy to observe the evolution of the γ’ precipitates and found that nanoscale γ precipitates act to slow the rate of coarsening of the larger γ’ precipitates at 800 °C but are dissolved after a certain time, after which coarsening then resumes as for a conventional γ-γ’ system.
Having established that hierarchical structure is the means to enhanced thermal stability, the researchers were then concerned with understanding the mechanism of how these stabilizing nanoprecipitates are formed and dissolved. Atom probe tomography provides local chemical information on the nanoscale and was used by the team to identify the supersaturation of cobalt, ruthenium and rhenium driving the formation of the hierarchical structure.
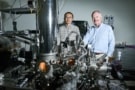
Grain dynamics make perfectly flat copper films impossible
Complementary modelling
To further explain the microstructural properties that result in mitigated coarsening, the scientists simulated the material structure using phase-field modelling. This enabled them to vary the composition of the material in order to calculate conditions optimized for the formation of a stable hierarchical structure, as well as measure the timescales and temperatures over which such a material would be stable. The model was consistent with the experimental findings, indicating a decrease in precipitate size at 800 °C is to be expected for times as long as 200 hours.
Making use of complementary microscopy and modelling methods is the key to developing and understanding the formation of materials capable of withstanding demanding environments. Based on these results, the researchers expect that alternative elements such as platinum may further increase the thermal stability of superalloys through the formation of more stable hierarchical nanostructures, bringing efficient manufacture of long-lifetime alloys one step closer. More details can be found in Science Advances.