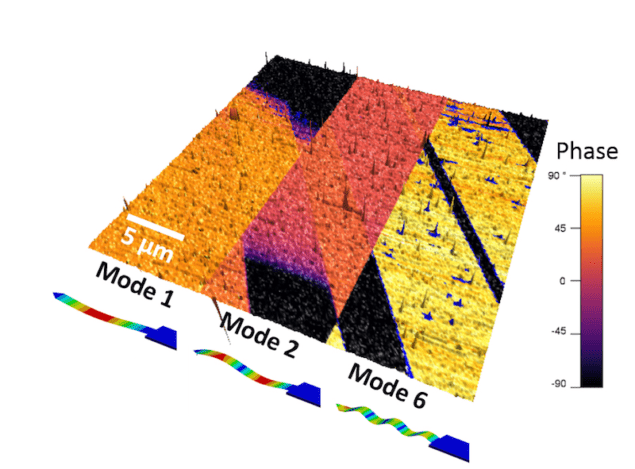
Piezoresponse force microscopy (PFM) and related strain sensing atomic force microscope techniques are a good way to characterize materials at the nanoscale. However, they do suffer from unwanted artifact signals that make the cantilever vibrate more. To reduce these vibrations, researchers normally use very stiff cantilevers but this means that PFM cannot be used to probe fragile materials, such as biomaterials and ultrathin films. A new technique that makes use of higher-order contact resonance eigenmodes (or resonant vibrational modes) could allow for much softer cantilevers to now be employed.
In PFM and related techniques, an AC voltage is applied between an atomic force microscopy (AFM) probe, or cantilever, and the sample being studied. When the surface of the sample electro-mechanically deforms, this causes the AFM probe to vibrate and these vibrations can be detected and measured. Until now, measurements were made almost exclusively at or below the so-called first flexural contact resonance frequency.
Such techniques are ideal for studying piezoelectric materials, which convert electricity into mechanical force. These include ferroelectric materials, perovskite photovoltaic active layers and even some biological materials. However, these measurements are prone to a number of artifacts that can produce paradoxical results. These artifacts come from electrostatic forces between the tip and sample, and electrostatic forces between the cantilever body and sample.
Stiff cantilevers can reduce these artifacts because they vibrate less, but their downside is that they destroy fragile samples.
Out with excessively stiff cantilevers
A team led by Jason Killgore of the National Institute of Standards and Technology (NIST) is now saying that higher-order contact eigenmodes can be readily excited in PFM and that these can improve the technique’s overall sensitivity, reduce electrostatic artifacts and increase the proportion of total signal coming from wanted, as opposed to unwanted, strains. “This means that you no longer need to use excessively stiff cantilevers and their correspondingly high forces to achieve artifact-free measurements,” explains Killgore.
“AFM cantilevers can exhibit an infinite number of eigenmodes at increasingly higher frequencies,” he tells nanotechweb.org. “As we excite higher eigenmodes, we in fact increase the dynamic stiffness of the cantilever by producing a number of nodes, which act as virtual supports along the cantilever length. And by increasing the dynamic stiffness we can nearly eliminate the effect of electrostatic forces between the sample and cantilever body, which are major sources of error in today’s PFM measurements, as mentioned.”
The researchers say they are thus able to use cantilevers that are a thousand times softer than those typically employed, and with improved force control, which means a fragile sample can now be studied. “We also see enhanced sensitivity to small surface strains and can tune our measurements to preferentially sense in-plane versus out-of-plane ‘inverse’ piezoresponse with a single cantilever,” says Killgore.
More complex vibrational shapes are a problem
The NIST team tested out its technique on periodically poled lithium niobate as a test sample. This material was chosen since it is routinely used in PFM studies.
It is not all plain sailing though. The researchers say that higher eigenmodes show more complex vibrational shapes compared to sub-resonance vibrations. This makes it complicated to determine the relationship between AFM cantilever amplitude and true surface displacement. “We are working on resolving this problem,” says Killgore.
The technique is detailed in Nano Futures 2 015005.