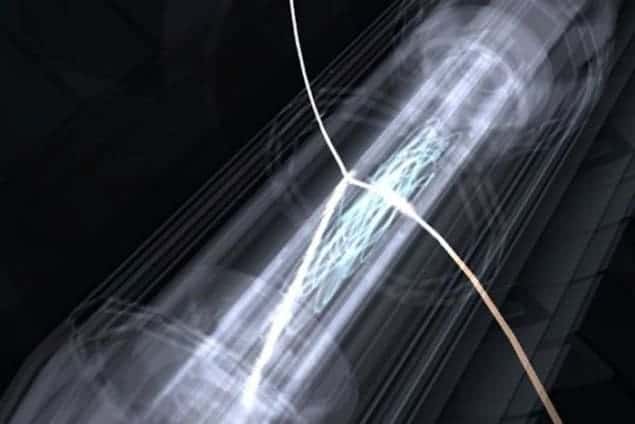
A method for laser-cooling magnetically trapped antihydrogen atoms to temperatures of about 20 millikelvin has been proposed by a team of researchers from Canada and the US.
The team claims that cooling the antihydrogen would make it much more stable and so easier to study in experiments. In particular, it could lead to better spectroscopic analysis of antihydrogen, so that its properties can be compared with those of hydrogen.
Antihydrogen is an atomic bound state of a positron and antiproton that was first produced at CERN in 1995. Over the past few years, physicists working on the ALPHA experiment at the Geneva lab became the first to capture and store a significant amount of the stuff, holding a total of 309 antihydrogen atoms for 1000 seconds in 2011. In early 2012 the team then showed that it is possible to probe the internal structure of an antihydrogen atom by carrying out the first tentative measurements of the antihydrogen spectrum. By improving such measurements, researchers hope to determine what structural differences, if any, antimatter has compared with ordinary matter. This, they hope, could eventually explain why the universe currently contains much more matter than antimatter.
Trapping dimensions
Most experiments such as ALPHA create antimatter by injecting positron and antiproton plasmas into a magnetic trap, where the antimatter is then held for some time and studied. But the antihydrogen atoms have relatively high energies compared with the depth of the trap. This causes distortions in the spectral analysis of the sample because of effects such as line-broadening.
To ensure that these effects do not affect experiments, Francis Robicheaux of Auburn University in the US (also a member of the ALPHA collaboration) and colleagues have suggested using “Doppler cooling” to reduce the energy of the trapped antihydrogen. The method uses laser light that is at a slightly lower frequency than a transition in an atom to slow the atom down. “As the antihydrogen cools, it moves more slowly and it stays near the centre of the atom trap. If the atoms are moving more slowly, the frequency shift because of motion – the Doppler shift – is reduced,” says Robicheaux, as he explains the benefits of the proposed cooling process. “Atoms travelling towards the laser would ‘see’ the light shifted up slightly and are more likely to absorb it than if they are travelling away from the laser. Thus, the laser tends to oppose the motion, which leads to slowing the atom down.”
The researchers need to use laser light at a wavelength of 121 nm (as it is slightly below a transition in antihydrogen), but creating a source that is intense enough is a challenge in itself. “It is not trivial to make the necessary amount of laser light at a specific wavelength of 121 nm,” says Robicheaux.
Three dimensions in one
The nature of the trap also poses a challenge. Robicheaux explains that most Doppler-cooling experiments use laser light coming from six directions in 3D, so that any atom in any direction has a laser that opposes its motion. But the ALPHA experimental apparatus only allows for laser light from one direction (so the cooling would be only in 1D). “Our calculation was meant to show whether the ALPHA experiment would get 1D or 3D cooling. The neat result from our simulation is that the atom motion is complicated enough that directly cooling from one direction still leads to cooling in 3D,” says Robicheaux. According to the team, the cooling could be carried out using either a continuous-wave Lyman-α laser (generally considered the laser of choice for antimatter cooling) or a pulsed laser, “as long as the intensity is high enough and the laser line width is small enough”.
Through a series of computer simulations, the team showed that antihydrogen atoms could be cooled to around 20 millikelvin, making the effort worthwhile. Currently, trapped antihydrogen atoms have energies up to 500 millikelvin.
Over the years, some other experiments and research groups have suggested similar cooling methods for antimatter. But the new proposal is the only one that uses one laser and the time required to do the cooling would be quite short – a matter of a few minutes. The researchers also say that the cooling should have no negative effects on the trapping efficiency. “There are good reasons to believe that cooling will increase the lifetime of the antihydrogen in the trap, but we have not yet done detailed calculations to prove it,” explains Robicheaux.
Optical versus magnetic trapping
Yasunori Yamazaki of the RIKEN laboratory in Japan, who was not involved in the work, feels that the new proposal contains important results and implications. “It is demonstrated by the simulation that the pulsed laser can cool antihydrogen down to 20 millikelvin, which is about a 10th of the trapped-hydrogen temperature now. In other words, the bad influence from the non-uniform magnetic field that is unavoidable when magnetically trapping antihydrogen can be reduced by a factor of 10, and so the spectroscopic accuracy can be improved by a factor of 10, which is great,” says Yamazaki. But he cautions that optical trapping of antihydrogen is essential to carry out very accurate spectroscopic studies, and how the cooling applies to that remains to be seen.
The researchers are now working on estimating just how much laser power would be needed to successfully carry out their suggested experiment.
The research is published in Journal of Physics B: Atomic, Molecular and Optical Physics.