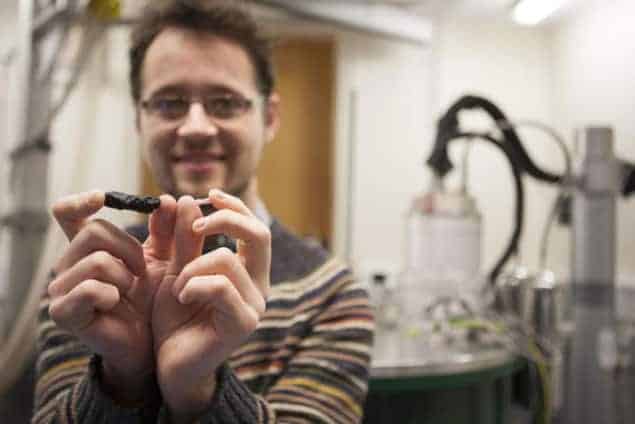
Solid wax models of “splash-form tektites” – tiny pieces of natural glass that are created when asteroids or comets impact the Earth – have been created in the lab for the first time by researchers in the UK. Using magnetic levitation to produce a state of weightlessness, the team created its own wax models of tektites that come in a variety of shapes – from spheres and elongated dumbbell-like shapes to doughnut formations. Geologists have long been keen to understand exactly how and when tektites form, but until now the shapes of the tektites have been derived solely from numerical simulations. The new experimental technique shows, for the first time, that the models are indeed correct.
When an asteroid or comet smashes into the Earth, a large, very hot, quantity of impact material is created and molten rock is ejected from the Earth’s surface. Tiny drops of this liquid spin and cool to form a natural glass before dropping back to the planet’s surface. Tektites are found mainly in Australasia, Central Europe, North America and the Ivory Coast – in areas associated with extraterrestrial impacts.
Spinning shapes
These droplets of splashed rock often have rotation imparted by the impact, and the shape of such spinning droplets as they fly through free space depends on the rate of spin. Rotation first deforms a droplet to create a flattened sphere. If it is spinning fast enough, the droplet assumes a more elongated shape, and as it becomes unstable it begins to pull apart and look like a dumbbell. Most importantly though, the tektites cool as they travel through the atmosphere, and so completely solidify into their final shapes before they fall to the ground. A fraction of those that survive the trip are studied by geologists to determine the type and age of the rock that was impacted. They are also used to correlate known impact sites and prehistoric extinction events, or to find impact sites that have not yet been discovered.
We could gain even more information from tektites if we understood exactly how they form in the air, but recreating such shapes in the lab is a daunting task. “There aren’t many materials that can hold molten rock, and even then you need to spin and cool a single droplet of it simultaneously,” says Kyle Baldwin of the University of Nottingham in the UK. Baldwin, together with Richard Hill, also from Nottingham, and Samuel Butler of the University of Saskatchewan in Canada, have come up with a way to do just that.
Levitated drops
The trio uses a superconducting magnet that has a strong enough field strength and field gradient to levitate diamagnetic materials – water, alcohols and wax – which are weakly repelled by magnetic fields. The researchers create their tektites by first melting wax on a hot plate, pipetting it into the magnetic field and spinning it up using carefully directed air flow. The floating wax is then allowed to cool and solidify into whatever shape it forms because of its rotation, thereby recreating some of the conditions that tektites experience as they form in the air.
Baldwin told physicsworld.com that the highly deformed shapes had only been calculated numerically using sophisticated computing and had never been measured directly by experiment. Indeed, others had tried to recreate tektites in the lab before, but in previous cases the liquid drops were either imaged while still in rotation (they never solidified) or were in contact with some kind of surface before they solidified, which affects their final shape.
“This is the major contribution of our work, to recreate the shapes of equilibrium and confirm, for the first time, that the shapes predicted by simulations are in agreement with the shapes made though experiment,” says Baldwin, pointing out that “computer simulations are only effective if they begin from an initial agreement with experiment and extrapolate forward into more complicated systems. Until now, this validation had been lacking.” Butler, who has carried out previous research on simulating tektite formation, provided supporting simulation evidence that the shapes created by the Nottingham researchers were indeed the actual equilibrium shapes of spinning liquid droplets. The team also found that above a certain droplet size (∼0.5 ml), the tektites wrinkled as they solidify – this may be similar to the surface wrinkling seen in natural tektites.
Ketchup or wax?
Thanks to the obvious difficulty of using actual molten rock in a lab, there exist a variety of analogues used by geophysicists who study the fluid mechanics of lava/magma flows. These include ketchup, honey and paraffin wax. Baldwin and colleagues chose the latter, for the simple reason that wax is solid at room temperature, and by heating it to a temperature of only about 55 °C, they could easily melt it and inject it as a liquid into the magnet bore without too much difficulty, while also giving them ample time to perform the experiment.
Splash-form tektites are one of four types that have been found. Because they are distinguished primarily by the idea that their shape is dominated by their rate of rotation, they can be perfectly recreated in the team’s experiments. “That, however, is not to say that this technique could not be extended to recreate the conditions found in the other types of tektites, such as aerodynamics,” says Baldwin. “One might imagine experimenting with, for example, the influence of air flow, bubbles, impact speed, etc to further examine the variety of conditions tektites are under as they form, but this is speculation at the moment.”
In the future, the team also plans on applying its experimental method to investigate rubble-pile asteroids, which are spun up by solar winds and deform into various shapes. These are so small that gravitational effects alone cannot explain how they hold together, but they are also too large for molecular cohesion to be sufficient.
The research is published in Scientific Reports.