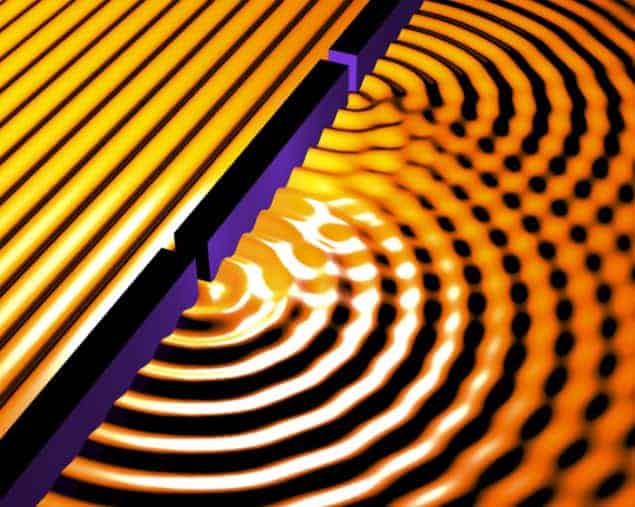
A new version of the famous double-slit experiment has allowed physicists in Israel to measure a phenomenon that is bizarre even by the counterintuitive standards of quantum mechanics. By placing a double-slit experiment along one path of a larger double-slit experiment, the researchers have shown that photons traverse a section of the apparatus that they neither enter nor exit. The effect, the team argues, is best understood by invoking a little-used interpretation of quantum mechanics that was first proposed in 1964.
Perhaps the simplest and starkest demonstration of wave–particle duality is the famous double-slit experiment. Particles such as photons or electrons that are emitted discretely behave as waves when they pass through two slits and build-up an interference pattern when detected individually on a screen.
In this latest version of the experiment, Lev Vaidman and colleagues at Tel-Aviv University used Mach–Zehnder interferometers as double slits and photons as particles. The optical interferometer uses a beamsplitter to divide the photon beam into two separate paths that are then recombined and sent to a detector. A difference in the lengths of the two paths dictates how the beams interfere when recombined, which affects the intensity measured by the detector.
Three possible paths
In the Tel Aviv experiment, an inner Mach–Zehnder interferometer is placed in one path of an outer interferometer so that the recombined beam continues its journey through the outer device and on to a detector (see figure below). This means that a photon has three possible paths from source to detector. The goal of the experiment is to find out which paths are taken by at least some photons arriving at the detector. This is called a weak measurement, and is consistent with the laws of quantum mechanics because it does not involve measuring the path of any specific photon.
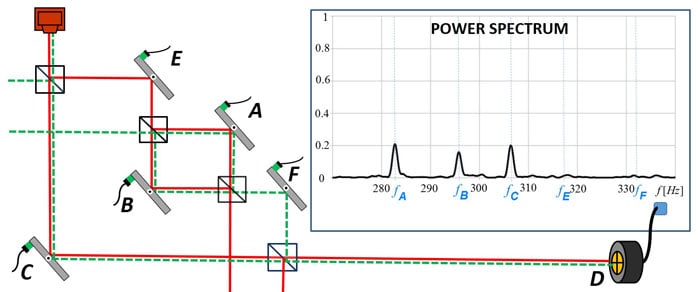
To make their measurements, the researchers set all the mirrors in the interferometer vibrating slightly, each at a different frequency. As a mirror vibrates, it alters the pathlength of any light reflecting from that mirror. This alters the phase difference when the beam is recombined, changing the intensity at the detector. As every mirror is vibrating at a unique frequency, oscillations in the detected intensity at a particular frequency indicated that photons have touched a specific mirror.
The researchers arranged the two pathlengths through the inner interferometer so that the two paths interfered destructively when they recombined. Therefore, no light could leave the inner interferometer. One might expect, therefore, that the only oscillation in the detected intensity would come from the mirror bypassing the inner interferometer, but this was not what the researchers found.
Bizarre conclusion
The detected intensity did indeed oscillate at the frequency of this bypass mirror, but it also oscillated at the frequencies of the mirrors in the inner interferometer. It did not, however, oscillate at the frequencies of the mirrors directing light into or out of this inner interferometer. This leads to the bizarre conclusion that some photons received by the detector had passed through the inner inteferometer, but had never entered it and never left it.
The researchers believe that this validates an unconventional interpretation of quantum theory called the two-state vector formalism. It was first proposed in 1964 by Yakir Aharonov, Peter Bergmann and Joel Lebowitz. Here, the probability of finding a particle in a particular place is the product of two vectors: one evolving forwards in time from the source and one evolving backwards in time from the detector.
A photon can touch a mirror if and only if both waves are non-zero at that point. The inner interferometer causes any wave leaving it to be identically zero. The forward-evolving wave is zero on the way out, and so no photons can be found here. The backward-evolving wave travels backwards through the interferometer and is therefore zero on the way in, so no photons can be found here either. Within the inner interferometer, however, both forward- and backward-evolving waves are non-zero, and so photons pass through both arms (see figure).
Intuitions and explanations
Vaidman stresses that the two-state vector formalism does not actually make different predictions from the conventional wave-mechanics approach devised by Erwin Schrödinger in the 1920s. However, the results of this experiment seem highly counterintuitive and are difficult to rationalize using the traditional method. “You can define constants and you can have intuitions about what is going on using the two-state vector formalism,” says Vaidman, “But it’s not something that standard quantum mechanics cannot explain in the end.”
Onur Hosten of the University of Illinois at Urbana-Champaign, who was not involved in the experiment, says that whether you consider the experiment using the two-state vector formalism or using the conventional wave-mechanics approach, the effect is generated by the fact that performing a weak measurement inevitably perturbs the system. Oscillating the mirrors does itself change the pathlengths, thereby destroying the perfect destructive interference between the two paths of the inner interferometer and allowing the wavefunction to leak out. The probability of a photon leaking out is effectively zero, however, because the probability is proportional to the square of the wavefunction, which tends to zero much faster than the wavefunction itself. “From my perspective, it’s really interesting to understand why you get the results you do,” says Hosten, adding “but it’s also interesting that a weak measurement gives you some disconcerting answers.”
The results are to be published in Physical Review Letters. A preprint is available on arXiv.
- Weak measurements are explained in detail in the article “In praise of weakness” by Aephraim Steinberg, Amir Feizpour, Lee Rozema, Dylan Mahler and Alex Hayat of the University of Toronto