Astronomers at the Event Horizon Telescope are setting up the most elaborate of shots, as they aim their global network of telescopes towards the black hole at the centre of our galaxy. Tushna Commissariat investigates how it is possible to image an object that swallows everything, including light
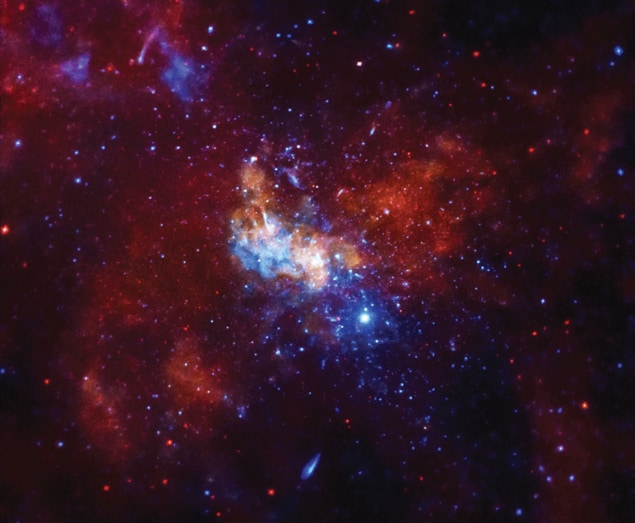
We live in the age of photography. The huge popularity of Instagram, Snapchat and the “selfie” suggests that the old idiom of a picture being worth a thousand words has never rung more true. It is no surprise then that this mania for photography has hit a team of tenacious astronomers, who want to capture something infinitely more exotic than the stars, galaxies and nebulae that the Hubble Space Telescope and its many successors regularly image.
The subject of these astronomers’ fancy though, is an elusive muse. Hidden behind colossal veils of gas and dust, some 26,000 light-years away, the tiny spot of their fascination is one of the most difficult things to image in the universe – a black hole. More specifically, they want to capture the four-million solar mass supermassive black hole that lies at the heart of our Milky Way galaxy, dubbed Sagittarius A* (SgrA*).
It sounds an impossible feat – after all, as its name suggests, a black hole is a point in space from which nothing, not even light, can escape. But that problem is not about to stop the researchers involved in the Event Horizon Telescope (EHT), who are determined to image SgrA* within the next few years.
Bright horizons
Supermassive black holes are thought to lie at the centres of most galaxies in the universe, and astronomers are keen to decipher their key properties – such as how these behemoths “eat”, how their extreme gravity affects the space–time around them, and how some of them fuel the massive jets of material that spew out from the galaxies that host them.
A black hole’s “event horizon” is the boundary at which even light cannot escape its gravitational pull, as the velocity required to do so would be greater than the speed of light – something forbidden by Einstein’s general theory of relativity. The theory – which celebrates it centenary this year – introduced the radical notion that space–time is dynamic and affected by matter. General relativity can be verified either on some of the largest scale structures in the universe – such as a galaxy supercluster – or in places where the effects of gravity are extreme. While the theory has passed many tests, the EHT researchers want to see just how well it holds up at the “ultimate proving ground” – a black hole’s edge.
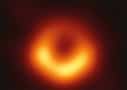
First images of a black hole unveiled by astronomers in landmark discovery
The impact of a supermassive black hole is felt across an entire galaxy, spread out over hundreds of thousands of light-years. But according to Sheperd Doeleman of the Haystack Observatory at the Massachusetts Institute of Technology (MIT), the real action – or more precisely, the gravitational structures that the researchers are keen on observing – happens very close to the black hole itself. According to Doeleman, who is the lead astronomer at the EHT, observing such structures as they change and evolve is one of the main motivations of the group.
While black holes may have a far-reaching impact and a gargantuan mass – the largest detected to date is thought to have a mass 17 billion times that of our Sun – they are relatively small; the biggest of them could fit snugly within the solar system. Although SgrA* is the black hole with the largest “apparent” size as viewed from Earth, thanks to its proximity to us, trying to visually resolve its structures is as challenging as spotting an orange on the Moon. “Imagine your friend is holding a quarter in Los Angles and you’re standing in New York, and now you can read the date on the quarter,” says Doeleman.
Despite their name, black holes are not all dark. The gas and dust trapped around them in an accretion disc is so compact that it is often heated to billions of degrees even before it is swallowed, making the objects glow brightly. Indeed, general relativity also predicts that a black hole will have a “shadow” around it. Thanks to the immense gravity at the event horizon, the light generated by heated in-falling gas will follow a hyperbolic trajectory – sometimes even looping back on itself instead of travelling in a straight line. The result is a shadow-like ring that encloses a dark centre. The light may warp in such a way that it takes a 180° turn, which would allow astronomers to study the far side of the object.
The shadow is of great interest as its size and shape depends mainly on the mass, and to some small extent the possible spin, of the black hole, thereby revealing its inherent properties. Theoretical predictions based on general relativity have already ruled out certain shapes – an egg-shaped shadow for example, is not on the menu. Seeing SgrA*’s actual shadow will therefore be “yet another consistency check for general relativity,” says Avery Broderick of the University of Waterloo and the Perimeter Institute for Theoretical Physics in Canada. Broderick, who is a theorist and part of the EHT team, has modelled what the shadow should look like. He told Physics World that the shadow’s appearance “encodes information about the surrounding space–time, acting like a CAT-scan of the environment”. These observations therefore “will set the stage for strong-gravity research”.
Directly observing SgrA*’s shadow, which is only 50 micro arcseconds across, is no mean feat. Astronomers would need a telescope with an angular resolution comparable to the event horizon; such an instrument would be roughly the same size as our planet, which is clearly impractical. The EHT astronomers will instead resort to a radio-astronomy technique known as very-long-baseline interferometry (VLBI), in which synchronized radio signals, from an astronomical source, are picked up by a network of individual radio telescopes and telescopic arrays scattered across the globe.
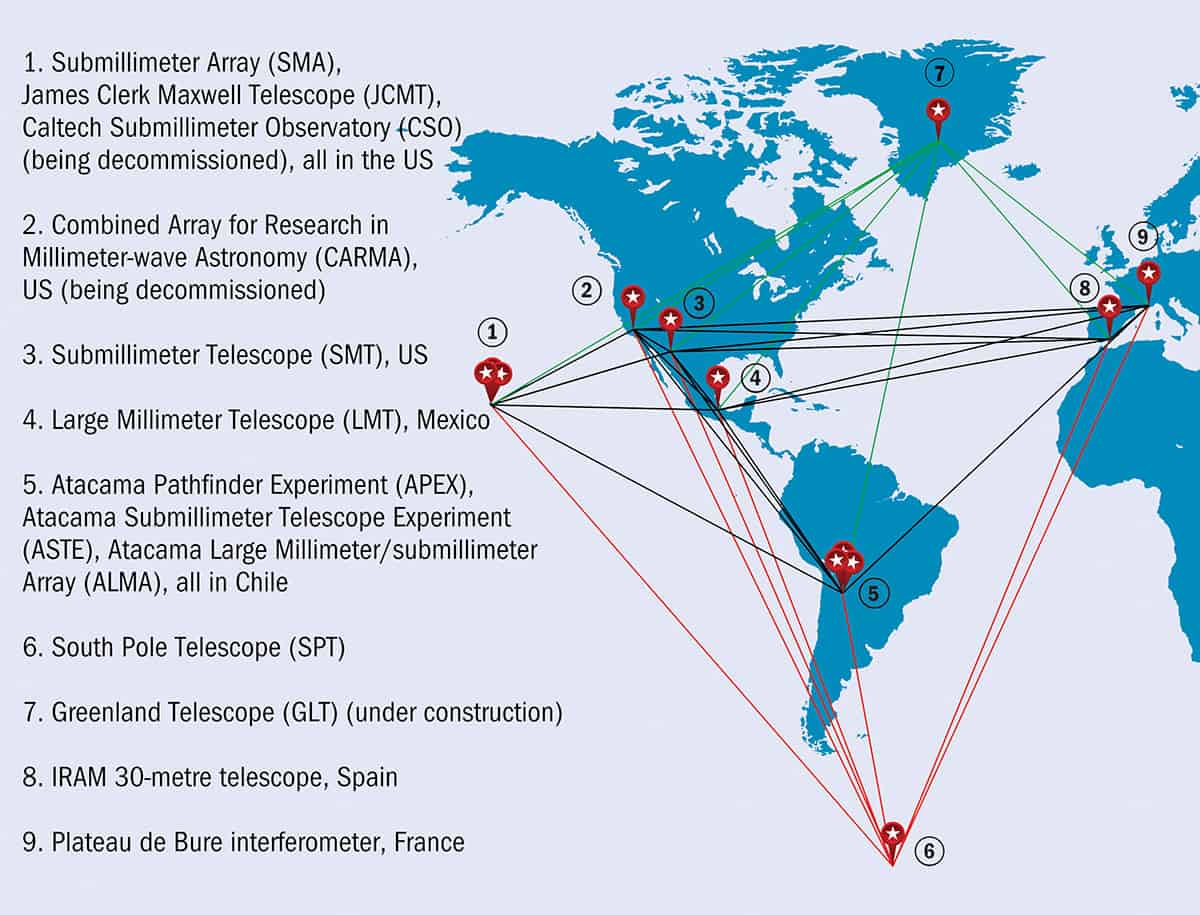
The distance between each pair of telescopes or facilities determines a “baseline”, and together these baselines effectively create a massive virtual telescope the size of a continent or larger. The signals received at each “antenna” (each individual telescope dish) in the network are precisely tagged with a very accurate time stamp, normally using an atomic clock at each location. The signals are later correlated and used to build up a complete image.
The technique lets astronomers build up an Earth-scale telescope “mirror”, with only little points silvered at the spots where each antenna lies. As a radio source is observed over the course of a night, the Earth’s rotation means that each silvered point spins out into a line, filling in enough of the mirror to make an image. The resolution that can be achieved using the VLBI technique is proportional to the observing frequency, which is at the submillimetre wavelength for the EHT. Early measurements have shown that it is possible to resolve some of SgrA*’s structures at 1.3 mm. According to Broderick and Doeleman, these observations confirmed that short-wavelength VLBI can be used to directly probe SgrA*’s event horizon. Each antenna in the EHT will eventually detect SgrA* at very high frequencies to penetrate the dust and haze that surrounds it.
Connecting the dots
For their first set of measurements in 2007, the EHT astronomers linked up the James Clerk Maxwell Telescope (JCMT) in Hawaii, the Submillimeter Telescope in Arizona and the Combined Array for Research in Millimeter-wave Astronomy (CARMA) in California. Since then, the team has successfully added the Atacama Pathfinder Experiment (APEX) dish in Chile, the South Pole telescope, the Institut de Radioastronomie Millimétrique’s 30 m dish in Spain, and earlier this year the Large Millimeter Telescope (LMT) in Mexico. Broderick points out that all of the individual facilities “are wildly different, so putting them all together has been a challenge”.
When the EHT becomes fully functional in the next few years, it will consist of telescopes and arrays that extend from Hawaii to Spain, all of which will study SgrA* simultaneously. This will make it the highest-resolution instrument on Earth, taking images with up to 2000 times better resolution than the Hubble Space Telescope. The improvement is largely due to technological advances made in the last two years, including a doubling of the radio-frequency space within which the EHT can image, which has made the entire array more sensitive. The number of telescopes in the array itself has also been nearly doubled, and will continue to grow once the Plateau de Bure interferometer in France and the Greenland Telescope (under construction) join the EHT.
What the EHT astronomers are most excited about this year is the successful completion of their five-year-long programme to upgrade the Atacama Large Millimeter/submillimeter Array (ALMA) in Chile to join the EHT. ALMA was designed so that each antenna pair creates a single baseline, the longest of which can be up to 16 km. For ALMA to be able to synch with the other EHT telescopes, the EHT team and the astronomers and engineers in Chile needed to turn ALMA into a “phased array”, such that its 66 individual antennae function as a single radio dish 85 m in diameter. In this kind of an array, the signals from each antenna are added together, which for ALMA requires adding specialized electronic equipment and computing abilities designed and built at the National Radio Astronomy Observatory in the US, the National Astronomical Observatory of Japan and MIT’s Haystack Observatory.
A key piece of technology added last year was a custom-built hydrogen maser that the EHT team installed, which allows ALMA’s data to be time-stamped so they can be processed with the other sites’ data. Following the upgrade, ALMA joined forces with APEX in January this year to create a 2.08 km baseline – a proof-of-principle test to check that ALMA can synch with the EHT by spring 2017 at the latest. ALMA and APEX simultaneously observed a quasar, “0522-364”, which is a very radio-bright source. ALMA successfully used its new maser to ensure both telescopes were truly in synch by time-stamping the data, which are now being processed at MIT. According to Doeleman, the successful observation marked “a huge step toward making first images of a black hole” with the EHT.
Beyond the veil
Once the EHT is fully ready, Doeleman hopes that astronomers will be able to do more than image SgrA*’s shadow and that they will be able to detect structural changes in the morphology of SgrA* in real time. Black-hole shadows were originally thought to be static, but according to Doeleman, our latest understanding suggests that they may vary, changing shape as the black hole accretes more mass.
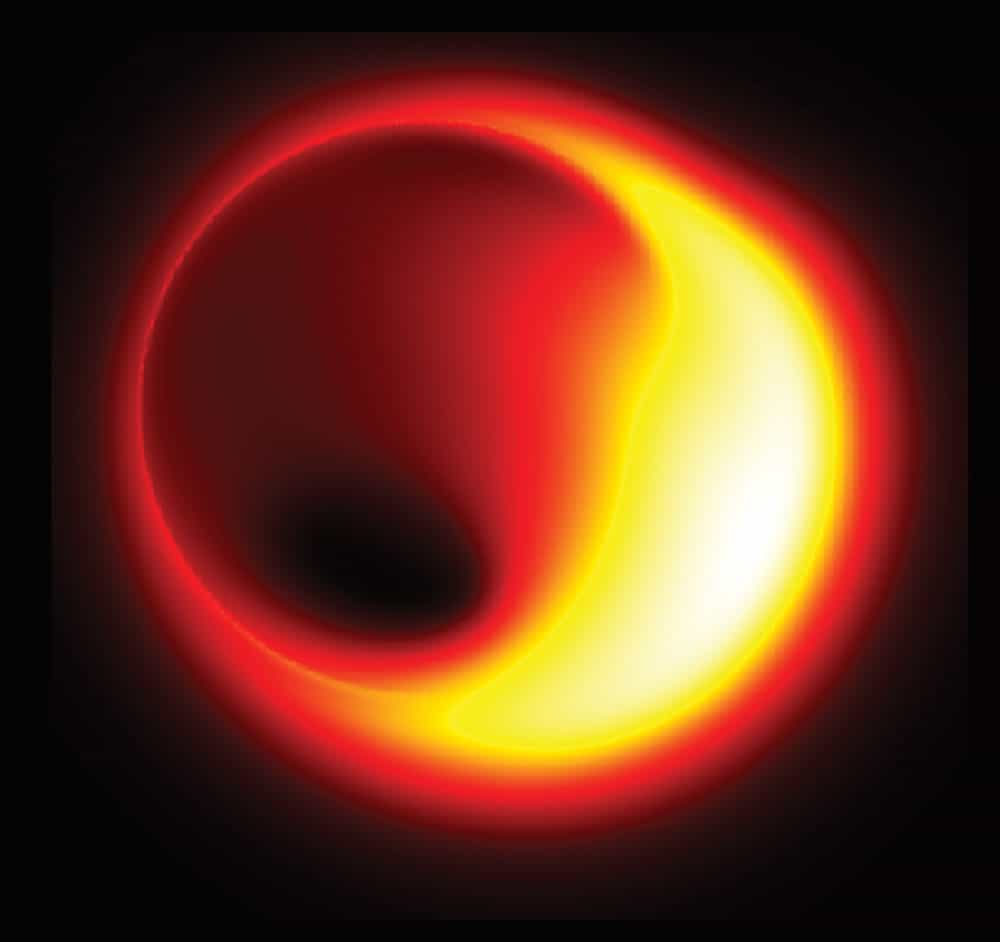
This, he says, was initially seen as a problem – their image could get “smeared out” when SgrA* is active (it is currently relatively quiet as there is not too much matter falling into it). But Doeleman realized that this potential bug could become a feature – even if the researchers cannot take an image when SgrA* is swallowing matter. By using triplets of the EHT’s antennae in combination, the astronomers could look for real-time changes in the accretion flow, thereby detecting what Doeleman describes as the object’s “very heartbeat”. They would then be looking at the evolution of a black hole’s structure, resolved in real time. This is surprisingly easy: if SgrA* is indeed a non-spinning black hole, as our current theories suggest, then matter trapped in its accretion disc should revolve around the hole once every half hour. If SgrA*is spinning, this number would drop to mere minutes for matter moving in the same direction as its spin, or increase to around five hours if the matter were moving in the opposite direction. Either way, this means that the EHT could potentially see structural changes several times during a single night’s observation – an amazing feat considering the vast timescales normally involved in astronomy.
Valued-added science
One of the main benefits of the EHT is that no new telescopes or arrays need to be built specifically for it. Instead, EHT astronomers simply book time on already existing telescopes and add some extra hardware and software (such as ALMA’s maser) where needed. Indeed, Broderick describes it as a “value-added project” as it is ultimately much cheaper than building a facility from scratch. Doeleman adds that they were purposefully slow and measured in building up the EHT’s current array – which has been on the drawing board since 2008 – by proving to staff at each site that they can deliver interesting results, thanks to the successful observations made to date.

Magnetic fields near the Milky Way’s black hole seen for the first time
Despite the benefits, coordinating with lots of people all over the world to determine policies and funding is tricky. Another problem is having to send experts to far-flung locations for each of the EHT’s observational runs, which means that the group members spend a lot of time travelling. They also miss the odd observational window when there are unexpectedly good weather conditions at all the sites. In the future, the group plans to put specific software into place at each site, such that the telescopes can be remotely accessed from a central location.
Data are currently recorded on custom-built high-capacity, high-speed hard-drives that are filled with helium and are hermetically sealed to stop them being damaged by the extreme conditions (such as low atmospheric pressure) where most of the telescopes are located. The data-filled drives at each location are then flown to centres in Europe and MIT to be processed. Doeleman explains that with so much data collected in a single night’s observation, air travel is surprisingly the fastest and most secure means of transmission. The team therefore has to quickly transfer the recorded data and send the drives back for the next round of observations. Doeleman hopes that they can make this process more efficient in the near future, especially when the number of data gathered will be up to 1 petabyte per site for each five-day observational session when the array is running at full capacity.
Adventure science
With the EHT researchers spanning the globe, they are currently transforming from an ad-hoc group to a real collaboration. And that progress is helped by a history of successful results and long-term collaborations with facilities like ALMA.
For Doeleman, the project embodies the spirit of “adventure science”, motivating team members as they scatter to high-altitude sites from Hawaii to Mexico all the way to the South Pole. “There is some faint echo, which reaches back across 100 years, to the kind of observations that were done to first vet general relativity,” he adds, referring to Karl Schwarzschild reportedly solving Einstein’s field equations in the trenches of the First World War, and Arthur Eddington who travelled to Brazil to observe the 1919 solar eclipse in a bid to confirm Einstein’s prediction that the light from stars close to the Sun would be distorted. “This is one of the aspects of the project that really resonates with people,” says Doeleman. “At least, that is what gets me going.”