
Grain boundaries in ceramics may not be as chemically stable as previously thought. So say researchers at the University of Wisconsin-Madison in the US who have found that carbon atoms collect or segregate at the boundaries of silicon carbide – a technologically important ceramic – when the material is exposed to ionizing radiation. The result could help improve our understanding of ceramics in general and aid the development of better ceramic materials for applications in nuclear energy.
Changes in the chemical composition of grain boundaries – the interface between two different grains in a polycrystalline material – can dramatically affect a material’s mechanical strength, corrosion resistance and radiation tolerance. This is because the boundaries act as traps for defects, sites for corrosion reactions and channels for diffusing other chemicals through the material.
Radiation-induced segregation
Radiation-induced segregation is a well-known phenomenon in metal alloys. When such alloys are irradiated, the bombardment of neutrons, ions and other particles creates defects. More specifically, defects known as Frenkel pairs form when an atom leaves its place in the crystal lattice (creating a vacancy) and lodges in a nearby location (becoming an interstitial). Frenkel pairs can then either recombine with each other or migrate to defect traps such as grain boundaries. If different types of atoms in the alloy move at different rates, certain elements build up or become depleted in the vicinity of the boundaries.
Because ceramics have stronger interatomic bonds than metals, researchers had long assumed that the atoms in ceramics were not subject to this type of segregation. Now, however, a team of researchers led by Izabela Szlufarska has turned this idea on its head by studying the behaviour of grain boundaries in silicon carbide (SiC). This ceramic is employed in nuclear energy and jet engines, among other high-tech applications, and it also shows promise for advanced nuclear reactors and microelectrochemical systems operating in harsh conditions.
Szlufarska and colleagues found that the material’s grain boundaries became enriched with carbon atoms when they bombarded it with ions at a temperature of 300°C. At this temperature, which is much lower than is required to initiate radiation-induced segregation in metals, they found that the radiation dislodged some carbon atoms from their normal lattice sites. Just as in metals, the resulting pair of defects in the SiC included a vacancy site and an interstitial (in this case, a loosely-bound carbon atom). They also observed that these untethered interstitials migrated towards the grain boundaries, where they accumulated, changing the material’s chemistry. The degree of segregation diminished when they irradiated the material at 600°C.
Radiation as a tool to fine-tune chemistry
The researchers obtained their result by analysing the chemical composition of grain boundaries in pristine samples of SiC grown by chemical vapour deposition using the latest scanning transmission electron microscopy techniques at UW Madison and Oak Ridge National Laboratory. They say that the phenomenon is likely to occur in other polycrystalline ceramics, too, and they also note that the segregation might be turned into an advantage, by making it possible to produce new types of ceramic materials with improved properties. “The radiation might in fact be used as a tool to fine-tune grain boundary chemistry,” explains study co-author Xing Wang.
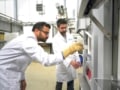
Salt and water cut ceramic processing costs
However, the researchers, who report their findings in Nature Materials, also note that the much lower temperatures for carbon enrichment in SiC suggest that our understanding of radiation-induced segregation in metals may not transfer directly to ceramics.
“Unlike metal alloys, ceramics have much more complex energy landscapes for defect reactions and multiple sublattices in which defects can migrate,” they say. To explore this landscape, the team developed an ab initio informed rate theory model that reproduces and explains the low-temperature radiation-induced segregation behaviour in SiC. These calculations also suggest that carbon segregation near grain boundaries in SiC comes about due to two factors: the different diffusivities of vacancies and interstitial defects; and the different reaction energy barriers between silicon and carbon sublattices in the material.
In their future studies, Szlufarska and colleagues plan to study how radiation-induced segregation in SiC depends on other conditions – for example, the total dose of radiation. They will also investigate different types of grain boundaries.