
A technique for addressing individual electronic and nuclear spins in a diamond crystal has been developed by researchers in Japan. The scheme combines optical and microwave processes and could lead to the creation of large-scale systems for the storage and processing of quantum information.
Electronic and nuclear spins in some solid-state crystals are promising platforms for large-scale quantum computers and memories. These spins interact weakly with their local environment at room temperature, which means that they can operate as quantum bits (qubits) that store quantum information for very long times. Furthermore, such spins can be controlled without significant losses. Typically, the spins respond to both optical light and microwaves. Optical light is good for spatial precision in addressing individual spins because of its shorter wavelengths. The longer microwaves, on the other hand, provide higher-fidelity control of all the spins in a crystal at the cost of no spatial resolution.
Now, Hideo Kosaka and colleagues at Yokohama National University in Japan have developed a way to address individual spins that combines the strengths of both optical and microwave control. They used microwaves to control individual spins in diamond by precisely “spotlighting” them using optical light. They demonstrated site-selective operations for information processing and generated entanglement between electronic and nuclear spins for information transfer.
Diamond NV centres
For its spins, the team used nitrogen–vacancy (NV) centres in a diamond crystal. These occur when two neighbouring carbon atoms in a diamond lattice are replaced with a nitrogen atom and a vacant site. The ground state of an NV centre is a spin-1 electronic system that can be used as a qubit to encode information.
To perform computation, one needs to be able to change the spin state of the qubits in a controlled manner. For a single qubit, it suffices to have a set of four cardinal operations to do this. These are the identity operation and the Pauli X, Y, Z gates, which rotate the state about the three axes of the Bloch sphere.
Universal holonomic gates
These operations can be implemented by using dynamic evolution, where a two-level system is driven by a field at or near resonance with the transition to “rotate” the qubit to the desired state. Another way is to implement a holonomic gate, where the phase of a state in a larger basis is changed so that it has the effect of the desired gate on the two-level qubit subspace. Compared to dynamic evolution, this method is considered more robust to decoherence mechanisms because the phase acquired does not depend on the exact evolution path of the larger state.
In this latest research, Kosaka and colleagues first demonstrate the site-selectivity of their technique by focussing a laser at a specific NV centre. This changes the transition frequency at that site such that no other site responds when the entire system is driven by microwaves at the right frequency. Using this technique, the team was able to spotlight regions a few hundred nanometres across, rather than the much larger areas illuminated by the microwaves.
By selecting sites this way, the researchers showed that they could implement the Pauli-X, Y and Z holonomic gate operations with good fidelity (greater than 90%). Gate fidelity is a measure of how close the performance of the implemented gate is to an ideal gate. They use a microwave pulse that flips its phase in between which makes the protocols robust to non-uniformities in power. They also show that a spin coherence time of about 3 ms is maintained even after gate operations that take a comparable time.
Quantum memories and networks
In addition to the electronic spin states, an NV-centre also has accessible nuclear spin states associated with the nitrogen nucleus. Even at room temperature, these states are extremely long lived because of their isolation from the environment. As a result, the NV-centre nuclear spin states can be used as quantum memories for storage of quantum information for long times. This is unlike qubits based on superconducting circuits, which need to be at sub millikelvin temperatures to overcome thermal noise and are more susceptible to decoherence caused by interactions with the environment.
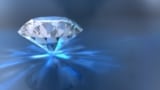
High-resolution diamond sensor maps electrical currents in the heart
Kosaka and colleagues were also able to generate entanglement between an electronic spin and a nuclear spin in the NV centre. This enables the transfer of quantum information from an incident photon to the NV centre’s electronic spin and then on to the nuclear spin quantum memory. Such a capability is critical for distributed processing where the photons can be used to transfer information between qubits in the same or different systems in a quantum network.
Writing in Nature Photonics, the researchers say that with modifications to their optical addressing process, it should be possible to improve its spatial resolution and also make use of coherent interactions between multiple NV centres. Combining a few different techniques could enable “selective access to more than 10,000 qubits in a 10×10×10 µm3 volume, paving the way to large-scale quantum storage”. Kosaka says that his group is now working on the challenging task of making two qubit gates using two nearby NV-centres.