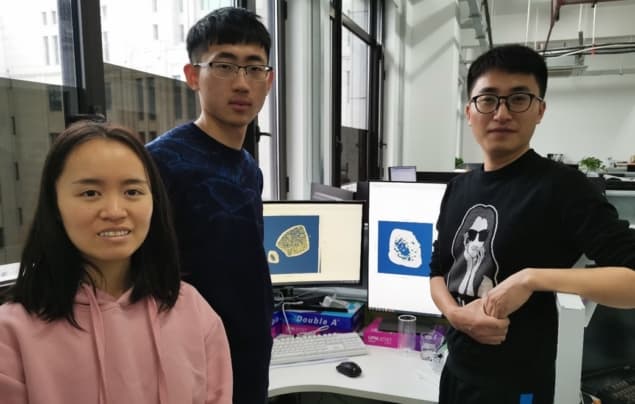
Osteoporosis is a bone disease characterized by the loss of bone mass and increased bone porosity. Such weakened bones are far more likely to fracture, making regular monitoring and early diagnosis of bone diseases essential.
The gold standard for evaluating bone status is dual-energy X-ray absorptiometry (DXA), which measures bone mineral density. But DXA is not suitable for quantifying other key mechanical parameters. Instead, ultrasound imaging could play a crucial role, with techniques such as ultrasonic computed tomography (USCT) able to characterize bone microstructure and biomechanical properties, as well as being inexpensive and non-ionizing.
Researchers at Fudan University in China have proposed a reconstruction algorithm that enables quantitative bone imaging using USCT. Writing in Chinese Physics B, they demonstrate the performance of their proposed method using a series of increasingly complex bone models.
Iterative approach
The main challenge when imaging bone with ultrasound is that the speed of sound in bone differs significantly from that of surrounding soft tissue; but common medical ultrasound techniques assume uniform sound velocity. Such methods cannot therefore accurately image irregular bone–soft tissue boundaries without prior knowledge of the sound speed distribution.
“Conventional ultrasonic B-mode imaging has inherent limitations in imaging biological hard tissue and bone using uniform sound velocity assumption,” explains researcher Dean Ta. “USCT provides a promising alternative. Particularly, USCT with full-waveform inversion shows potential for high-resolution bone imaging.”
Full-waveform inversion (FWI) is an image reconstruction algorithm originally developed for geophysics. In this study, Ta and colleagues employed frequency-domain FWI (FDFWI), an inverse process that reconstructs parametric images by minimizing the mismatch between measured and numerically simulated signals. When used with USCT, the FDFWI algorithm iteratively updates bone material parameters – sound velocity and mass density – in a simulated model until it reaches the best match.
The algorithm solves the inverse problem by calculating parameters at gradually increasing ultrasound frequencies, and then uses the final values to create quantitative bone images. “The FWI starts from a relatively low frequency to avoid ‘cycle skipping’ and ensure the iteration is not trapped in a local minimum,” Ta explains. “By gradually increasing to a high frequency, it can obtain a high-resolution inversion for bone structure.”
In theory, using a maximum frequency of 2.5 MHz, FDFWI can image pores and trabeculae in bone tissue with a spatial resolution of around 0.6 mm.
Computational models
To demonstrate the validity of their approach, the researchers estimated parametric bone images via FDFWI using a ring-array ultrasound transducer. They first modelled a simple 2 mm-thick tubular bone phantom with known mass density, using FDFWI to estimate the sound velocity. During the simulations, the ultrasound frequency increased from 100 kHz to 3.5 MHz in 100 kHz steps.
As the frequency reached 1.5 MHz, both the outer and inner edges of the phantom could be observed. At 2.5 MHz, the image was clearer with few artefacts, showing that FDFWI can accurately restore the macroscopic morphology. Increasing the frequency to 3.5 MHz made little further improvement.
Next, the researchers modelled a distal fibula (lower leg bone). Here, they used the FDFWI algorithm to simultaneously estimate sound velocity and mass density, using ultrasound frequencies from 100 kHz to 3.5 MHz in 50 kHz steps. They note that using this smaller interval ensures enough low-frequency components to reconstruct mass density.
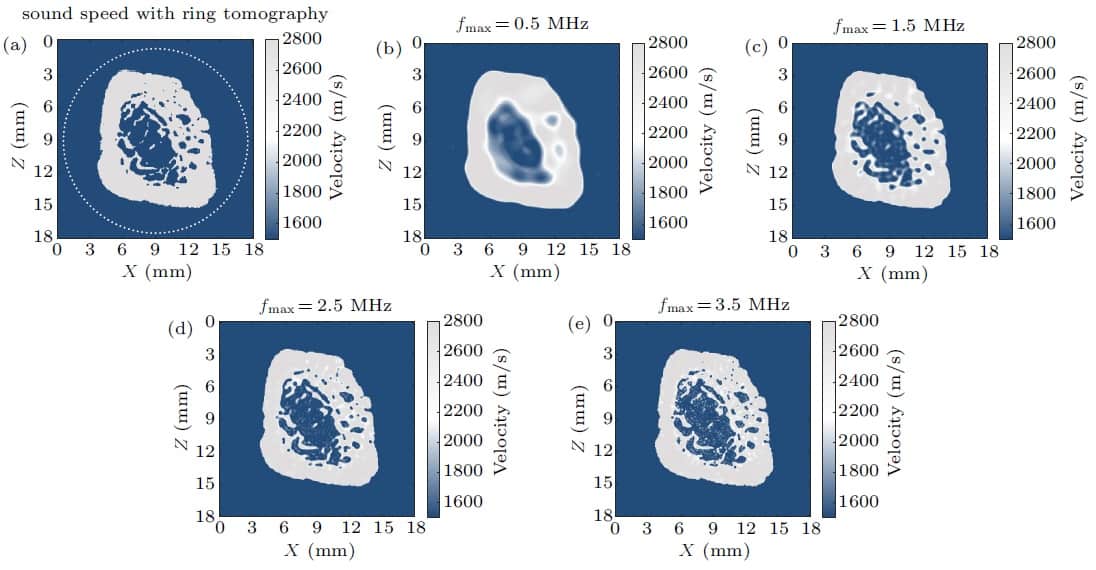
As the frequency gradually increased to 1.5 MHz, both the outer and inner edges of the velocity map were accurately recovered and microstructures in the bone could be clearly seen. At 2.5 MHz, the velocity map became clearer and some more subtle features appeared, demonstrating that FDFWI can accurately recover geometry and microstructure and provide high-resolution bone images.
The third numerical model used a distal tibia–fibula pair model derived from a high-resolution peripheral quantitative CT image. The FDFWI algorithm used ultrasound frequencies from 100 kHz to 2.5 MHz in 50 kHz steps. Even in this challenging scenario, FDFWI reconstructed both the macroscopic morphology and the microstructure with sub-millimetre resolution. Compared with the true CT image, the simulations clearly and accurately presented pores and trabeculae in bone images.
The researchers note that reconstruction of the density map was not as good as that of the velocity map. The reconstruction errors of both sound velocity and mass density were larger than seen in the single fibula model, attributed to multiple scattering and diffraction effects between the two bones.
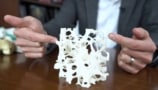
Breaking the mystery of bone micro-architecture
Finally, to investigate the robustness of FDFWI against noise, the team added random noise to the synthetic data generated in the tibia–fibula pair to create cases with signal-to-noise ratios of 30, 10 and 0 dB. They found that sound velocity maps could still be recovered well in the presence of noise. Even in the 0 dB case, the bone geometry and some relatively larger microstructure could be reconstructed.
The next step is to verify the effectiveness of the method using experimental rather than synthetic data. “We are trying to perform experimental studies on the topic of musculoskeletal system imaging with full-waveform inversion,” Ta tells Physics World.