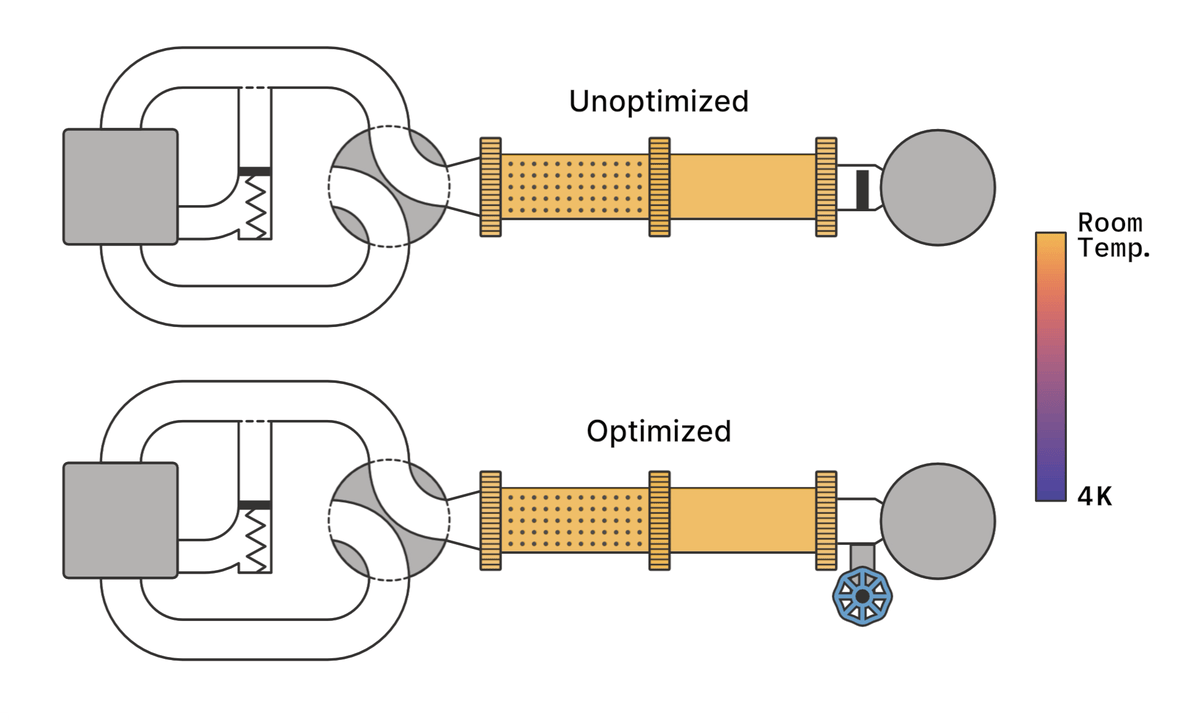
A simple modification to a popular type of cryogenic cooler could save $30 million in global electricity consumption and enough cooling water to fill 5000 Olympic swimming pools. That is the claim of researchers at the National Institute of Standards and Technology (NIST) and the University of Colorado Boulder who describe their energy-efficient design in Nature Communications.
Ryan Snodgrass and colleagues in the US have designed a new way to operate pulse tube refrigerators (PTRs), which compress and expand helium gas in cooling cycle that is similar to that used in a household refrigerator. Developed in the 1980s, PTRs can now reach temperatures of just a few Kelvin, which is below the temperature that helium becomes a liquid (4.2 K).
While PTRs are reliable and used widely in research and industry, they are very power hungry. When Snodgrass and team looked at why commercial PTRs consume so much energy, they found that the devices were designed to be efficient at their final operating temperature of about 4 K. At higher temperatures, the PTRs are much less efficient – and this is a problem because the cooling process begins at room temperature.
Easier repairs
As well as using lots of electricity to cool down, this inefficiency means that it can take a very long time to cool objects. For example, the Cryogenic Underground Observatory for Rare Events (CUORE) – which is looking for neutrinoless double beta decay deep under a mountain in Italy – is cooled to a preliminary 4 K by five PTRs in a process that takes 20 days. Reducing such long cooling times would make it easier and less costly to modify or repair cryogenic systems.
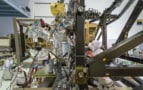
The ten-billion-dollar gamble: Keeping the JWST cool
A careful study of the room-temperature operation of PTRs revealed that the helium gas is compressed to a very high pressure. This causes a relief valve to open, sending some of the helium back to the compressor. Less helium is therefore used for cooling, reducing the efficiency of the PTR.
Snodgrass and colleagues solved this problem by replacing the manufacturer-supplied needle valves in a PTR with customized needle valves that can be adjusted constantly. These needle valves control the flow of gas between the refrigerator and its helium reservoirs. They are normally set to optimize the operation of the PTR at cryogenic temperatures.
In the new operating protocol developed at NIST, the needle valves are open at room temperature. This allows gas to flow in and out of the reservoir, which moderates the pressure in the refrigerator. As the temperature drops, the valves are slowly closed – keeping the system at an ideal pressure throughout its operation.
The team found that the modification can boost the cooling rate of PTRs by 1.7–3.5 times. As well as making cooling quicker and more energy efficient, the new design could also be used to reduce the size or number PTRs needed for specific applications. This could be very important for applications in space, where PTRs are already used to cool infrared telescopes such as MIRI on the James Webb Space Telescope.