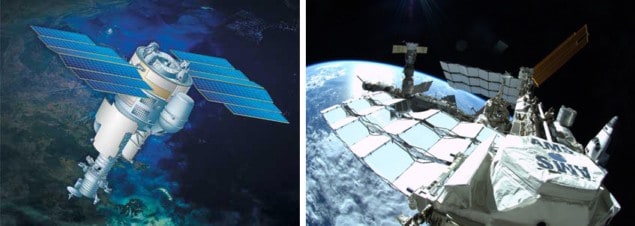
The positron “excess” measured by two independent space missions and linked by some physicists to dark matter or pulsars does not exist, according to new theoretical work done by an international team of researchers. Instead, the researchers have calculated a “robust” upper limit for the positron flux created via interactions of high-energy cosmic rays with ambient gas in the galaxy and say that the flux measured by the Payload for Antimatter/Matter Exploration and Light-nuclei Astrophysics (PAMELA) experiment and the Alpha Magnetic Spectrometer (AMS) lies below this limit.
Unexplained excesses
Positrons are the antiparticles of electrons. In 2008 the PAMELA collaboration released exciting data from its satellite, suggesting that cosmic rays above the Earth’s atmosphere contain an excess of high-energy positrons. The result was interesting because standard cosmic-ray theories suggested that the positron fraction should drop with increasing energy. But what was really exciting was that the excess could be the first evidence of dark-matter particles annihilating. However, doubts were cast over the initial PAMELA results and it was thought that the satellite could be confusing positrons with the far larger numbers of protons reaching its detectors.
Then in 2011 NASA’s Fermi Gamma-Ray Space Telescope confirmed PAMELA’s positron excess, followed by the AMS collaboration in April this year. Subsequently, the PAMELA collaboration has presented a new analysis of its data that confirms the excess. There are two popular explanations for this excess – one is that annihilating dark-matter particles are generating high-energy electrons and positrons and the other is that the positrons are being created in pulsars.
Plotting cosmic rays
But now, Kfir Blum and Boaz Katz at the Institute of Advanced Study at Princeton in the US and Eli Waxman at the Weizmann Institute of Science in Israel are arguing that there is no real positron excess. They say that the positron observations are well within their calculation of an upper limit of the number of high-energy positrons created when cosmic rays collide with ambient galactic matter. Indeed, the researchers suggest that the current positron measurements shed new light on the physics of cosmic-ray propagation – not dark matter or pulsars.
In their study, the researchers consider what they call the simplest reliable model to explain the positron flux. Their source is high-energy or “primary” cosmic rays that interact with gas and other matter that abounds in the galaxy. Blum tells physicsworld.com that this theory allows them to accurately calculate the amount of positrons that are produced in these “secondary” collisions and that many other species of particles, such as antiprotons and nuclei such as borons, are produced in these collisions. “While we can easily calculate the source of the positrons, predicting their actual flux at the Earth is harder,” says Blum. To make such predictions, a robust theory that explains how cosmic rays propagate in the galaxy is necessary and that is what is currently lacking.
Poorly understood propagation
Blum claims that this is a complicated theoretical problem that is not yet properly understood. “Lacking a reliable theory of the propagation of cosmic rays, the best we can do for positrons – reliably – is to predict a robust upper limit for their flux. The positron flux measured by PAMELA and AMS02 lies below this upper limit, and is consistent with it so there is no excess,” he says. Blum further explains that most of the claims of a positron “excess” are based upon speculative theoretical models of cosmic-ray propagation and since the problem itself is poorly understood “these models, more often than not, use many simplifying assumptions. When one tries to get to the bottom of the acclaimed ‘excess’, the issue always boils down to one or a few of these underlying simplifying assumptions, that may simply not be correct in the real world”.
If future data end up showing a positron flux above our limit, this could mean that an exotic source is needed. I for one would be thrilled. But this is not where I’d put my money in light of the data we have now. Kfir Blum, Institute of Advanced Study
The team also points out that it has applied its methods to antiprotons that are also produced in cosmic-ray fluxes. The difference between these calculations lies in the fact that antiprotons do not change their energy as they travel through the galaxy, while positrons do. “As a result of this simplification, for antiprotons we can compute precisely their actual flux [instead of an upper limit],” says Blum, explaining that the team uses this fact to validate its calculations. “Indeed, the measured antiproton flux agrees nicely with our prediction,” he says. In fact, the researchers have previously done similar upper-limit calculations for the 2009 PAMELA data, and say that the subsequent confirmation with AMS-02 only adds support to their theory.
Confined times
When asked if the new theory can explain why the positron fraction is rising with energy, Blum says it may be yet another hint of an interesting feature of how cosmic rays propagate. “As we show in our paper, one way (though not the only one) to obtain this kind of behaviour would be if the confinement time of the cosmic rays in the galaxy decreases with increasing energy – more rapidly so than the time it takes an average secondary positron to lose a significant fraction of its energy.” If this is indeed the case, then what the experiments are witnessing with the positrons merely amounts to rapidly falling confinement times of the cosmic rays.
Blum says the team’s theory can be tested experimentally in several ways. First, upcoming AMS-02 positron data at even higher energies will continue to check whether the observed flux remains below their robust upper limit, or not. “If future data end up showing a positron flux above our limit, this will rule out the secondary interpretation immediately. This would be wonderful news as it could mean that an exotic source is needed. I for one would be thrilled. But this is not where I’d put my money in light of the data we have now,” says Blum. He also explains that future AMS-02 measurements of secondary radioactive cosmic-ray nuclei, such as beryllium and aluminium isotopes will add independent information on the cosmic-ray confinement time. “This can then be contrasted with the positron data, to find out if we have a consistent picture. These radioisotope data are promising, as they could test for a failure of the secondary source hypothesis even in the case where the upper limit on the positron flux is not violated.”
Supernova source?
“What would have been more interesting is if they had been able to answer the question: what is the actual source of the ‘secondary origin of cosmic-ray positrons’?” says Subir Sarkar, a particle theorist at the University of Oxford in the UK who was not involved in the current work. Sarkar says his group’s research has shown that the source may be nearby supernova remnants that are accelerating high-energy protons, which interact with the ambient matter to make positrons that are also accelerated. “The prediction we made for the positron fraction – based on independent measurements of the positron/electron flux by the Fermi satellite – are consistent with the AMS-02 findings,” says Sarkar.
While Blum and colleagues admit that it is, in principle, possible that pulsars or dark matter may contribute to the experimentally measured flux, he insists that there is a good, if not definitive, theoretical reason to doubt the presence of a significant contribution from such an additional exotic source. “It would be quite surprising if a completely unrelated physical process, such as dark-matter annihilation, contributed to the positron flux in just the right amount to make the observed flux agree so well with the pure secondary prediction, neglecting energy loss,” concludes Blum.
The research is published in Physical Review Letters.