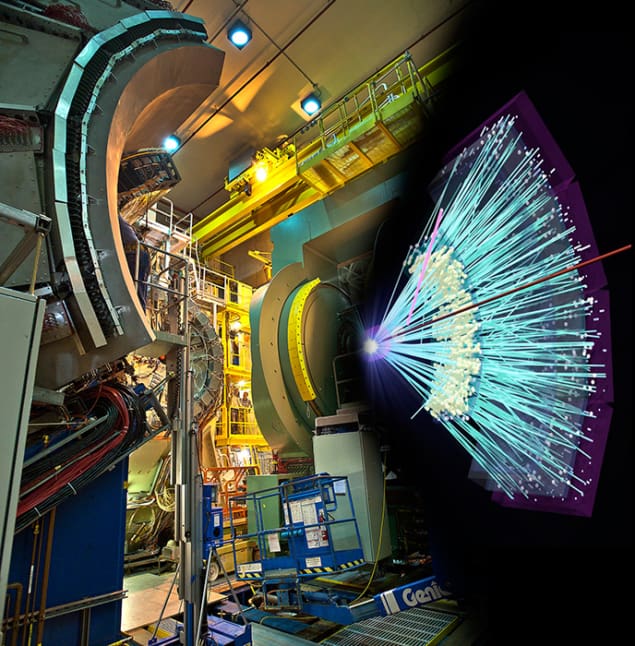
A tiny drop of an exotic ultra-hot “soup” that permeated the universe for an instant immediately after the Big Bang appears to have been created in collisions between gold nuclei and deuterons at the Relativistic Heavy Ion Collider (RHIC) at Brookhaven National Lab in the US. Evidence that a quark–gluon plasma (QGP) may be generated even in collisions involving very light nuclei such as the deuteron first emerged five years ago from data at the Large Hadron Collider in Geneva. But the new RHIC results push this evidence to record-low collision energies, which should help physicists better understand how a QGP forms and evolves.
A quark–gluon plasma (QGP) occurs at a temperature about 100,000 times that at the centre of the Sun. Protons and neutrons “melt” into an unbounded mass of quarks and their force-carrying particles, gluons. This extreme state of matter is believed to have persisted for only a few milliseconds after the Big Bang but can be created artificially by colliding very heavy nuclei that are travelling near to the speed of light.
The first, albeit indirect, evidence for such collisions was claimed by scientists at CERN in 2000, who smashed high-energy beams of lead ions into fixed targets made of lead or gold. Five years later, physicists working at RHIC also showed that they could create a QGP – in this case by colliding two beams of gold ions head on. However, these results contained a surprise: physicists had expected a QGP to behave like a gas, but instead it appeared to resemble a liquid.
Perfect liquid
Plotting the trajectories of the many thousands of particles created in each collision, the RHIC researchers found that the particles were not emitted in random directions as they would be in a gas. Rather, some of the emissions were correlated, meaning that more particles flew off at a narrow angle to the plane of the collision than were emitted at roughly right angles. This elliptical distribution signified that the particles were moving collectively and in fact “flowing” together like an almost friction-free perfect liquid, according to the researchers.
The latest work shows that this liquid state appears to be created even when heavy nuclei collide with very light nuclei at low energies. The first, unexpected, glimpse of such a small-scale QGP came in 2012 from the analysis of collisions between lead nuclei and protons at CERN’s Large Hadron Collider – events that were intended simply as a control for lead–lead collisions rather than to generate the scalding plasma themselves. That result prompted scientists in RHIC’s PHENIX collaboration to re-analyse data from collisions between gold nuclei and deuterons, particles consisting of one proton and one neutron. As they reported in 2013, they too saw telltale signs of fluid flow. A couple of years later the PHENIX team saw similar behaviour in collisions between nuclei of gold and helium-3.
Now, the PHENIX researchers have returned to gold–deuteron events. But this time they have studied the effect of varying the collision energy to see if the liquid behaviour disappears. Carrying out measurements over five weeks last year, they set the collision energy at four different levels: 200, 62.4, 39 and 19.6 GeV. They found very similar correlations at all energies when comparing the trajectories of particles grouped into pairs and into sets of four. At 200 GeV, they also looked at six-particle correlations. Their conclusion: liquid flow probably occurs all the way down to at least 20 GeV.
Tiny volumes
According to PHENIX deputy spokesperson Julia Velkovska of Vanderbilt University in Tennessee, the droplets they have created this time around are about 100 times smaller than those generated in collisions between large nuclei – occupying the volume of just four protons. Calculations suggest that the plasmas also only last for about a fifth of the time – a fleeting 7 ×10–24 s. “We have found correlations on scales much smaller than previously thought possible,” she says. “That means that as long as we achieve a high-enough energy density, size is not so important. A QGP may still be formed.”
The researchers’ next step is to see whether other expected signatures of a QGP, already verified in collisions with heavier nuclei, are also present in these smaller-scale collisions. One such signature is the abundance and flow pattern of different types of particles emitted in the collisions. Collaboration member Darren McGlinchey of Los Alamos National Laboratory says that abundance data provide information on the temperature of a QGP.
PHENIX colleague Ron Belmont of the University of Colorado says it is still possible that the elliptical emission they have observed is due not to the formation of tiny QGPs but instead down to nuclear properties prior to collision. When accelerated close to light speed, time slows down for the heavy nuclei, which means, according to quantum chromodynamics, that they appear as a dense wall of gluons. The fact that these condensates are thicker in the centre of the nuclei might explain why particles generated in the collisions are not emitted in random directions, he says.
Theoretical predictions
The expected effects of these condensates on the gold–deuteron collisions are still being worked out by theorists, says Velkovska, and as such have not yet been compared to the latest results. In contrast, she notes, predictions from hydrodynamics are in, and, she says, “agree with the data well”.
The results have been posted to the arXiv server (arXiv: 1707.06108 and arXiv: 1708.06983) and have been submitted for publication in Physical Review Letters and Physical Review C.