Neil Beattie describes some of the economic and political factors that impact developments of renewable energy materials and technologies besides the cost of crude oil
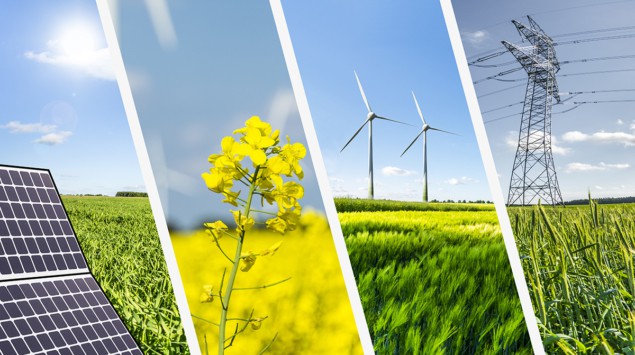
Unprecedented economic growth (2000–2008)
In August 2006 the price of crude oil peaked at just over $76/barrel having risen steadily through the early part of the decade. At the same time, global financial markets had bounced back from the dot-com crash of 2000 and despite the relatively high oil price, at least three all-business class airlines operated daily scheduled flights from London to New York. For business, these were the good times.
They were also good times for alternative energy because companies based in fossil fuels were reinvesting a significant proportion of revenues from core business, back into research and development (R&D). In the early part of the decade, the oil majors had investment portfolios that included solar, wind, hydrogen and biofuels. BP and Shell in particular developed successful photovoltaics (PV) businesses, manufacturing modules based on crystalline Si and thin film CuInSe2 respectively. Although by the mid-2000s, the cost of electricity from solar PV was still prohibitively high, prompting both BP and Shell to exit solar PV, they continued to focus investments in renewables including wind and – a sector naturally aligned to hydrocarbon supply chains – biofuels.
There is perhaps no better illustration of this period of exceptional economic growth than the march of the price of crude to an all-time high of just over $145/barrel in July 2008, at which point the global economy crashed spectacularly and crude dropped to under $50/barrel in just six months. As the ramifications of the global financial crisis (GFC) emerged, the oil price reflected investor uncertainty towards the end of the decade. With such uncertainty, the oil majors divested significantly to focus on core, large-scale oil and gas projects. This included in 2010, Shell’s exit from the London Array wind farm, which at the time was the world’s biggest wind project. The future for renewable energy seemed bleak.
Step forward renewables: A UK case study (2008–2018)
In the UK, government policy in response to the GFC was a reduction in public spending. Yet while other sectors suffered from funding cuts, the government continued to deliver on a commitment to introduce a financial incentive scheme for renewable energy technologies known as feed-in tariffs (FiTs). These had been successful in other countries such as Germany, and provided a key mechanism to achieve linked sustainability targets to curb CO2 emissions mandated by the government and the European Union. Informed by PV system performance research at Northumbria University, the FiT set for domestic PV installations offered an attractive return on investment for consumers of 10‑20% over the course of 25 years depending on installation costs. This was in stark contrast to savings rates on the high street that, after factoring in inflation, offered a negative return on investment. The cost of the FiT scheme was in fact borne by energy suppliers, which in turn passed this on to consumers. As a result, under strong political pressure to reduce the cost of energy bills, the UK government acted swiftly to ensure this window of opportunity lasted only 18 months by halving the FiT rate in December 2011.
Nevertheless, the overall result of the FiT scheme was to increase the installed capacity of domestic PV installations in the UK by two orders of magnitude in just over two years from its introduction. In other words, an increase of ~350,000 systems mostly on the roofs of houses. This remarkable growth in domestic PV installations in the UK is only one part of a solar story in the UK, which resulted in PV contributing a quarter of the total electricity supply on a sunny day in May 2017. Growth in ground-mounted solar farms in the 5–25 MW range kicked in early in 2013, slightly later than domestic systems most likely as a result of the longer incubation times associated with more complex planning and grid connection issues. Ultimately, these projects became increasingly attractive large-scale investments due to falling PV module prices. By 2018 they added approximately 4 GW of supply, which is around a third of today’s overall PV capacity of around 12 GW.
For wind energy the story is just as compelling when one considers that in the UK alone, 29.3% of electricity generation came from renewable energy fuel sources in 2017, and exactly half of this generation was from wind turbines. This demonstrated strong growth from 2010 when wind energy contributed approximately 2.5% of electricity supply, and provided a key contribution to energy security while meeting emissions targets. Similar to solar PV, the main driver of this growth was also the introduction of financial incentive schemes. Notably, although wind energy essentially remains subsidized in this market, solar PV is moving rapidly towards being subsidy-free while, crucially, remaining competitive.
The bigger picture (present)
In contrast to the PV electricity share in the UK, globally this figure stands at just 1.7%, indicating plenty of untapped market potential. History tells us that this figure is strongly sensitive to local subsidies which for example, appear to have worked well in Germany and the UK but not in Spain where an over-generous incentive scheme ultimately resulted in a solar tax. In that case, those who installed renewable energy technologies were ironically penalized because the incentive scheme rapidly became unaffordable.
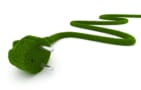
100% renewable electricity is viable
The Spanish case aside, the biggest story in solar today is that the levelized cost of electricity (LCOE) from PV is now competitive with fossil-fuel based sources. The driver behind this trend is Chinese PV manufacturers, who continue to sell modules at barely more than the cost of raw materials despite European and US anti-dumping policies. Some of these companies have posted very recent profit warnings that, if reproduced across the sector, will result in price stabilization or even rises. However for now a notable consequence of the drop in the LCOE from PV, alongside the Paris Agreement of 2016, is the market re-entry of both BP and Shell with recent investments in solar farms, including BP’s Lightsource and Shell’s Silicon Ranch.
New technologies and sustainability challenges (2018–2030)
So what do the past 18 years tell researchers working on advanced materials for energy? First and foremost, we need to be realistic and accept that for large-scale power generation, cost is fundamental. In 2018, the PV market was 95% crystalline silicon. This is sustained by the growth of large-scale solar farms, which have continued to drive down PV module prices through economies of scale to the extent that they may be considered almost a commodity product.
Despite their success so far, these PV modules are not necessarily suitable for new applications enabled by massive proliferation in distributed and interconnected devices because of scale limitations and the reliance on conventional, less versatile semiconductor wafer processing fabrication. Tesla had some success in achieving miniaturization with their Tesla solar roof tiles, which look like regular tiles but operate as solar cells. However although they created a lot of excitement on their release in 2016, they are seemingly not cheap or quick to install, and so far uptake has been limited. That said if efforts to overcome issues of scale and fabrication are successful, in sectors such as transport and healthcare PV modules could offer benefits beyond basic power generation. For example, they could provide complementary or back-up power sources that reduce range anxiety for electronic vehicle users, facilitate ultra-long flight for low-orbit Earth observation, power small-scale portable devices and generally provide a safety net for devices primarily fuelled by other means. The combination of offering new benefits at a smaller, more distributed scale, would mean that PV doesn’t have to compete with fossil fuels on a purely $/kWh basis. PV technologies with new properties and functionalities such as thin film and perovskite, which can be synthesized in solution on flexible substrates, could then compete more effectively.
Analysis…reveals a relative insensitivity to the oil price but a sensitivity to subsidization
An additional factor in recent PV competitiveness is appropriate integration with battery storage. For example, the recent installation of a 10 MW solar farm in Bedfordshire UK without subsidy was enabled by the installation of a 6 MW battery. There can be no doubt that the development of lithium batteries is enabling new applications such as electric aerotaxis (where correct PV-battery systems integration is vital) but there is an important distinction from PV materials: lithium is approximately 10,000 times less Earth-abundant than silicon. Comparison with indium suggests that although Earth-abundance will be important for cost, it may not be prohibitive. Indium has been commercialized successfully in high-value products such as indium tin oxide displays, despite being 100 times scarcer than lithium. Toxicity is potentially a larger barrier to commercialization and has been identified as a strategic issue for sustainable innovation by the European Commission.
Most commercial solar PV modules are certified to the International Electrotechnical Commission’s requirement of ≤0.8% degradation per year for 25 years, which defines a natural timescale for replacement. This means that new, high-efficiency PV technologies based on materials innovations for example in nanotechnology, must be proven with a distinct value proposition as early as 2030 in mature markets where widespread replacement may occur within a relatively limited period. However with materials sustainability also now a key driver, researchers must add the lifecycle of an energy material to performance criteria. Replacement of PV modules also presents specific recycling challenges and while the aluminium frames have clear and accessible value, encapsulated high-value elements such as silver-coated tabbing wires are technically more challenging and energy intensive to recover. Nevertheless, this is an area that offers opportunities for innovation and is not unique to a particular PV cell technology. Similar to PV modules, a typical wind turbine can be expected to be in operation for 20–25 years, and replacement in a sector that has seen enormous recent expansion will generate significant quantities of materials excess. Again, some components (masts, nacelles) will be compatible with existing recycling supply chains but turbine blades, which are glass or carbon fibre composites, are not. New and economic separation processes and approaches that target the interface between materials will be increasingly valuable.

The road to sustainability
Analysis of the past 18 years in renewable and sustainable energy reveals a relative insensitivity to the oil price but a sensitivity to subsidization. Too little incentive makes it difficult to achieve critical mass, while too much incentive defeats the objective. However, by the time effective subsidies are introduced, technologies are a long way from the laboratory. Electric vehicles are an example application where critical mass has not yet been achieved (although global leader Norway is the exception with approximately 39.2% market penetration) but although subsidy levels may vary from country to country they will universally apply to technology that is lithium based. This is challenging for energy materials researchers particularly in the context of funding objectives that are increasingly linked to economic prosperity.
In the near term, the materials innovations that have the greatest chance of success are those that play to new and niche markets in generation and storage, together with those that enable energy efficiency and recovery of high-value materials through viable recycling. In the long term, certain constraints will become more relaxed because the provision of new benefits, such as autonomous battery recharging, will provide a competitive advantage for PV technologies that are currently too expensive or have too short a shelf-life to compete with silicon. Ultimately, those who can convert energy cost competitively at the point of use have a strong proposition.
Acknowledgements
The author is particularly grateful to Alex Savidis of Narec Distributed Energy for useful discussions and colleagues at NUPV.