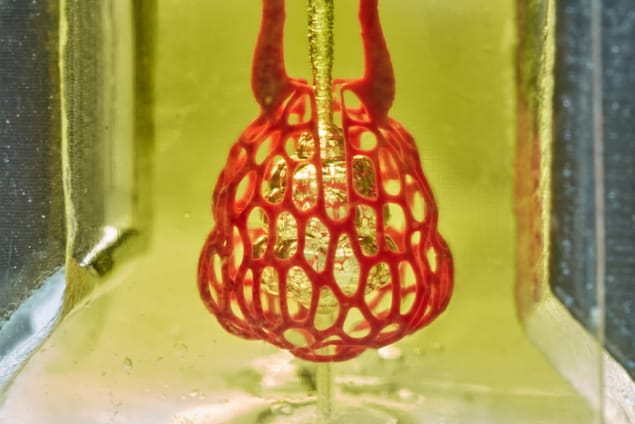
An unexpected ingredient – food dye – could be used to 3D print biomaterials that contain intricate, physically intertwined networks similar to the vasculature in native tissue. This is the new finding from researchers at Rice University and the University of Washington in the US who have used the technique to make tissue that mimics the structure of the lung and liver-like tissue that they have successfully transplanted into mice.
One of the goals of 3D bioprinting is to engineer tissue and organs in the laboratory. In this technique, 3D objects are printed layer-by-layer according to a pre-programmed design using liquid or semi-liquid bioinks (hydrogels) containing living cells. The perfusable structures produced are kept in a bioreactor that supplies them with nutrients so that they can develop into mature tissue.
Although researchers have already managed to print a variety of biocompatible tissues using 3D bioprinting, it is proving difficult to engineer vascular networks in engineered biomaterials using the method. Vascular networks are responsible for carrying nutrients to tissues and organs and examples in the body include bile ducts and blood vessels in the liver and airways in the lung.
One way of creating complex vascular bioarchitectures is using a 3D printing method called projection stereolithography, which converts photoactive liquid resins into structured solid materials via photopolymerization reactions. Although a highly efficient technique, the light-blocking chemicals employed to stop the polymerization are unfortunately highly genotoxic carcinogens and thus cannot be used in biofabrication.
Non-toxic photoabsorbers
A team led by Jordan Miller of Rice University and Kelly Stevens of the University of Washington have now discovered that common food dyes – both synthetic and of a natural origin – can make for powerful biocompatible photoabsorbers and be used to produce complex and functional vascular networks within hydrogels.
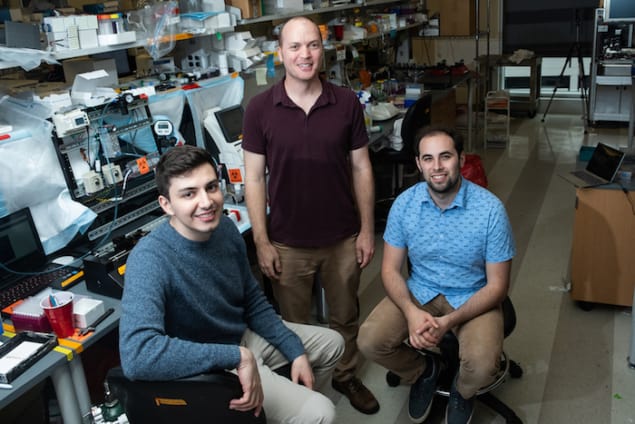
The researchers found that aqueous pre-hydrogel solutions containing tartrazine or E102 (which is yellow), curcumin (from turmeric) and anthocynanin (from blueberries) can be used in this context.
In this work, Miller and colleagues focused on tartrazine. They created a new open-source bioprinting technology, the ‘stereolithography apparatus for tissue engineering’ or SLATE, to test out their technique, which prints layers of hydrogels from a liquid pre-hydrogel solution that solidifies when exposed to blue light. As each layer solidifies in turn, an overhead arm in the printing apparatus raises the growing 3D gel by just the right amount so that a very fine layer of liquid is exposed at each light exposition step.
By adding a food dye that efficiently absorbs light in this wavelength range, the technique can produce biocompatible gels with an intricate internal architecture in just minutes, says Miller. Such a short time frame is advantageous for live cells that do not survive very long and which need to be put back in a perfusion culture as quickly as possible.
Lung-mimicking structure
The team, which also includes bioengineers from Duke University, Rowan University and Nervous System in Massachusetts, made a structure that mimics a lung and found that it was robust enough to withstand pulsatile “breathing” (or tidal ventilation and distension) – an intake and output of air that simulates the pressures and frequencies of human breathing. The red blood cells in the structure could take up oxygen as they flowed through the vasculature in the breathing air sac, in a way that is similar to the gas exchange that takes place in the lung’s alveoli.
“We were very interested in the architecture of the lung because it is such an incredibly complicated design and really challenging from an engineering viewpoint,” says Miller. “Although we can mathematically describe alveolar morphology as 3D space-filling tessellation (or tiling) of polyhedra, making such structures in the lab is no easy task since it requires ensheathing vasculature that closely encompasses the curvature of the 3D airways.”
To overcome this problem, Miller and colleagues developed a new bioinspired alveolar model that mimics this vasculature in the so-called Weaire-Phelan topology that contains both convex and concave regions resembling natural alveolar sacs with a shared airway atrium supporting alveolar buds. They extended the air surface in the structure in the normal direction and removed faces and ensheated edges in a smooth polygonal mesh to form a highly branched vascular network containing 185 vessel segments and 113 fluidic branch points that encompasses the airway and follows its curvature. This work was done in collaboration with researchers at Nervous System.
Liver-like construct
The team also printed 3D tissues and loaded them with primary liver cells aggregates before successfully implanting them into mice with chronic liver injury. The tissues are multi-material and have separate compartments for blood vessels and liver cells.
“The liver is a particularly interesting organ to be able to biofabricate since it is the largest solid organ in the body and carries out hundreds of crucial tasks that depend on its structural topology,” says Stevens. “There is currently no machine or therapy that can replace all these functions when it fails.”
“Our dye-based bioprinting techniques can also produce intravascular features such as bicuspid valves that allow for unidirectional fluid flow,” explains Miller. “In humans, such valves are also found in the heart, leg veins and networks like the lymphatic system that lacks a pump to drive flow.
“By creating multi-vascular and intravascular structures, we’re introducing an extensive set of design freedoms for engineering living tissue and we now have the freedom to build many of the intricate structures found in the body.”
Indeed, Miller, Stevens and study lead author Bagrat Grigoryan say they are commercializing key aspects of their research through a start-up company, Volumetric, which is based in Houston.
Form and function
“The form of a biological structure is an evolutionary consequence of its function,” Miller reminds us. “As evolution advances, we get more refined structures so the question we asked ourselves in this work was: can we reproduce this relationship in bioengineering by building more complex structures?”
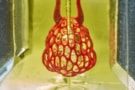
Food dye helps 3D-print vascular networks
The team, reporting its work in Science 10.1126/science.aav9750, is now using its (freely available) 3D-prinitng design to produce even more complex structures than those thus produced. “The idea is to look at the unit cell structures of different tissue, and then build an interconnected structure that better mimics real tissue,” Miller tells Physics World.
“We would like other groups to pick up our tech, even if they utilize some future 3D printing technology that doesn’t exist today. We believe that 3D bioprinting, which has been around for over 40 years already, will be a crucial aspect of medicine in the next 20 years,” he states.
- Read our special collection “Frontiers in biofabrication” to learn more about the latest advances in tissue engineering.