
A new imaging technique gives scientists the 3D position of individual atoms within an optical lattice for the first time, surpassing previous methods that provide only 2D images. Developed by a team at the University of Bonn, Germany and the University of Bristol, UK, the technique could improve the precision of atom-based quantum simulators and aid the development of new quantum materials.
“We are now able to take a single snapshot of the atoms in an optical lattice and see exactly where they are in all three dimensions,” explain Carrie Weidner and Andrea Alberti, who co-led the technique’s development. “Previous optical detection techniques were limited to taking ‘flat’ pictures of the atoms, but atoms do not live in a flat world.”
Experiments on atoms in optical lattices typically begin by using laser light to cool the atoms to temperatures just above absolute zero. This slows them almost to a halt and allows them to become trapped in a standing wave of laser light – the lattice. Once trapped, the atoms are exposed to an additional beam of laser light that makes them fluoresce. By imaging this fluorescence, researchers can determine the atoms’ position.
This imaging process is known as quantum gas microscopy, and it was developed more than a decade ago by physicists at Harvard University in the US and at the Max Planck Institute of Quantum Optics in Germany. The standard method, however, provides only the x and y coordinates of each atom. Information on the atoms’ position in the z direction – that is, their distance from the objective within the imaging system – was lacking.
Phase changing
The new method remedies this by taking the light emitted by the fluorescing atoms and modifying it before it reaches a camera. More specifically, the method changes the phase of the emitted light field so that the atom image appears to rotate in space as a function of its position along the imaging system’s line of sight.
“Instead of the typical round specks usually produced in quantum gas microscopy, the deformed wavefront produces a dumbbell shape on the camera that rotates around itself,” Alberti explains. “The direction in which this dumbbell points is dependent on the distance that the light had to travel from the atom to the camera.”
The dumbbell thus acts a bit like the needle on a compass, allowing researchers to read off the z coordinate according to its orientation, adds Dieter Meschede, who leads the Bonn laboratory where the experiments took place.
An idea with a long history
According to Weidner, the original idea for the study came from William Moerner and Rafael Piestun at the universities of Stanford and Colorado, respectively. Alberti adds that it is “fascinating” that no one else had previously thought of using the phase of the light field to obtain information about the z-position of the light-emitting particle. Controlling the phase of the light field is certainly not new, he says.
“It has actually a long history: in fact, to obtain sharp (and not blurry) images, all well-designed imaging systems are constructed to make the phase of all light rays reaching the camera surface (or the retina in our eyes) the same – this is the famous Fermat’s principle,” he explains. “Equalizing all of these phase differences is what corrects optical aberrations. This is essentially what we do when we wear eyeglasses to improve our vision.”
One of the biggest challenges with the technique, Alberti adds, was finding a capable experimenter who could work full-time to bring it to fruition. “We were lucky that Tangi Legrand, a master’s student, decided to take on this challenge,” he says. “Without him, we would not be reporting on our successful results today.”
Precise locations with a single image
Being able to precisely determine the 3D positions of atoms with a single image could be useful in several contexts. It could make it easier to trigger specific interactions between atoms, and it might help scientists develop new quantum materials with special characteristics. “We could investigate the types of quantum mechanical effects that occur when atoms are arranged in a certain order,” Weidner suggests. “This would allow us to simulate the properties of three-dimensional materials to some extent without having to synthesize them.”
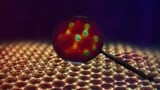
New technique puts 3D quantum gases under the microscope
A further advantage is that the technique, which is detailed in Physical Review A, is very general. “Our method can be applied to many systems, including molecules, ions, really, any quantum emitter,” Weidner says. “We hope to see this method applied in 3D quantum simulation efforts around the world.”
In the longer term, the researchers say their “dream” is to reconstruct the 3D positions of large arrays containing several thousand atoms. These large arrays require a large field of view, which entails optical aberrations, they explain. “We hope that improved reconstruction methods will be able to deal with these aberrations and therefore extend the field of view over which our technique can be applied,” they say. “They might also help find the 3D positions of atoms located above each other in more densely filled lattices.”