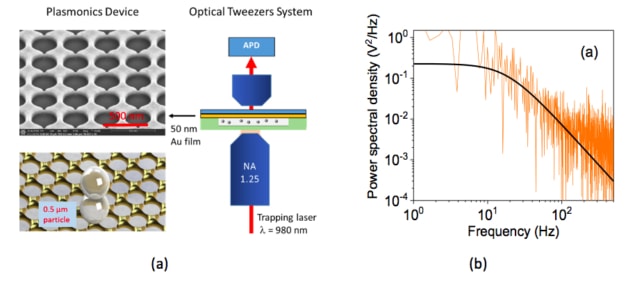
Researchers in Japan have developed new plasmonic tweezers that can gently capture and trap micron- and submicron-sized particles at specific locations in a fluid. The devices, which work in the near-infrared wavelength region using low-intensity incident laser light, could be integrated into lab-on-a-chip devices that can trap and transport biological cells.
Being able to manipulate nanoscale objects in liquid environments is one of the main goals of modern nanotechnology. Researchers usually do this by trapping particles with optical, acoustic, magnetic, electric or flow fields, and such technologies have led to breakthroughs in biophysics and microfluidics in recent years. However, the problem is that it is difficult to control objects that are sub-micron in size using these techniques since the trapping force decreases with the size of the object.
Plasmonic tweezers, which work by exploiting the localized electromagnetic fields near metallic nanostructures, are a solution to this problem because they can trap objects that are subwavelength in size. Indeed, researchers have already succeeded in trapping particles that are 10 nm in diameter or smaller using these devices. They have also made plasmonic tweezers based on large arrays to optically transport dielectric micron and submicron particles across a chip.
Another advantage of these devices is that their resonance frequency can be tuned towards the near-infrared (NIR) part of the electromagnetic spectrum. This frequency does not photodamage trapped particles nor does it heat them up – something that is particularly important for biological samples.
Multiple electric field “hot spots” trap particles
In their work, researchers led by Nic Chormaic of the Light-Matter Interactions Unit at the Okinawa Institute of Science and Technology Graduate University developed a noble metal annular aperture array in which they can simultaneously generate multiple dipole-like plasmonic resonances. These resonances produce multiple electric field “hot spots” that can trap particles. “We used this configuration to increase the density of trapping sites and therefore improve the trap stiffness of the particles over the surface of the annular apertures,” explains Viet Giang Truong, who is lead author of this study.
“We fabricated the nano-apertures on 50-nm-thick gold coverslips (from PHASIS Geneva, BioNano) using a focused ion beam (FIB) milling method,” says Truong. “We used a modified Thorlabs optical tweezers kit (OTKB) to trap particles and packed the plasmonic chip in a sample cuvette containing 0.5 μm and 1 μm polystyrene microparticles in deionized water. A Ti:Sapphire laser, with a focused beam diameter of about 1 μm and a wavelength tuned from 940 to 980 nm was used for the trap.”
The researchers used their plasmonic tweezer arrays to gently trap and transport micron- and submicron-sized particles to an assigned location at non-damaging low trapping intensities (< 1.5 mW/μm2) in the NIR wavelength region. They say that the devices could be integrated into lab-on-a-chip devices to trap and transport large particles such as biological cells using low incident power and a trapping laser frequency that can be tuned.
“In a recent extension of this work, we have shown that we can sequentially trap individual 30-nm-diameter polystyrene particles in multiple trapping sites of a similar plasmonic nanoring array,” Truong tells Physics World. “This study will appear in the journal Photonics Research (a preprint is already available at arxiv.org/abs/1805.01585).
“Based on these experimental results, we wish to develop a compact and user-friendly plasmonic nanotweezers systems that will allow us to sort specific particles, such as infected cells, from a mixed population. Such a device could not only be used to confirm the presence of these infected cells but also to trap, control and obtain genotypic type information through the binding processes of trapped proteins and molecules in the samples being tested.
The present research is detailed in the IOP journal Nano Futures 2 035007.