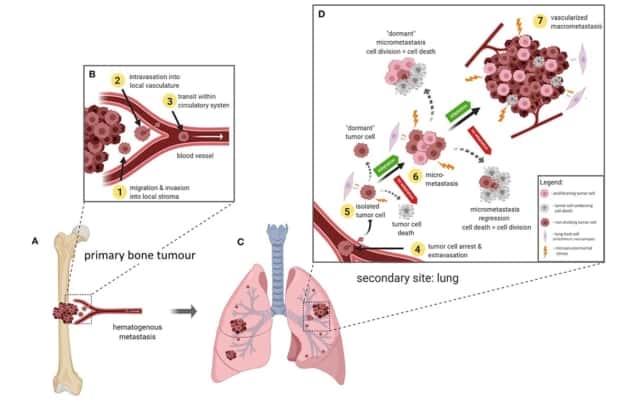
Accounting for the composition and mass of tumours could make dose calculations for radiopharmaceutical cancer therapy significantly more accurate, say researchers in the US. Edmond Olguin and colleagues, at the University of Florida and Radiopharmaceutical Imaging and Dosimetry, modelled how various radionuclides deposit energy in tumours of differing shape, size and tissue mineralization. They found that doses calculated for small tumours in bone are especially sensitive to such properties. The researchers say that their results should increase the effectiveness of radiopharmaceutical therapy, and are ready to be adopted by clinicians immediately.
While most forms of radiotherapy target individual tumours with precisely applied doses of radiation, radiopharmaceutical therapy is used to treat widely disseminated cancers by administering a radionuclide-labelled pharmaceutical to the patient as a whole. By choosing the right radionuclide, or by conjugating it to a certain drug, clinicians can make the radioactive agent accumulate selectively in tumours. This maximizes the dose to cancerous tissue while keeping the radiation burden elsewhere in the patient below harmful levels.
The most accurate way to calculate the received dose is to simulate the process using a radiation-transport model and a personalized digital phantom of the patient. But because this technique requires expertise and computational resources not available to most hospitals, it is most commonly used in research settings.
Instead, clinicians tend to use the Medical Internal Radiation Dose (MIRD) schema, a simpler method that classifies parts of the body as either radiation sources (where the radiopharmaceutical accumulates) or radiation targets (where there is a medical reason to measure exposure). In tumours that concentrate the radiopharmaceutical, these regions are one and the same.
When a single tumour is both source and target, the key to calculating the delivered dose is to determine the absorbed fraction – the proportion of energy emitted within the tumour that is deposited without escaping the region. This gives the dose per radionuclide decay, or “S-value”, which varies according to the type of particle emitted, its energy and the size of the tumour. Writing in Physics and Medicine and Biology, Olguin and colleagues show that the S-value is also sensitive to the tumour’s elemental composition, which until now has typically been modelled as soft tissue.
To investigate the effect of different tumour compositions, the researchers used a supercomputer to run the Monte Carlo N-Particle Transport (MCNP) model. They simulated photon, electron and alpha-particle irradiation of spherical and ellipsoidal tumours of various sizes and axial ratios, and with compositions ranging from 100% soft tissue to 100% mineral bone.
Considering a spherical tumour composed of soft tissue, the team found that their calculated absorbed fractions matched previous estimates to within a few percentage points for all radiation types and energies. For mineralized tumours smaller than 1.5 cm across, however, the absorbed fraction deposited by electrons was 25% larger than for soft-tissue tumours. For photons, the effect was even greater, with fully mineralized tissues absorbing as much as 71% more radiation than their unmineralized counterparts across all tumour sizes.
Olguin and colleagues also found that ellipsoidal tumours could be approximated as spheres, to a certain degree. Although electron absorbed fractions for spherical tumours agreed with their ellipsoidal counterparts within 8%, for photons, these errors were especially large, exceeding 20% for tumours with ellipticities greater than about 0.98.
Because dosimetric errors are greatest in small, mineralized tumours, the researchers expect their study to be particularly useful in treating patients suffering from the metastatic spread of the bone cancer osteosarcoma. To help get their results into the clinic quickly, they compiled a look-up table presenting S-values for a range of tumour sizes and compositions and a comprehensive list of relevant radionuclides.
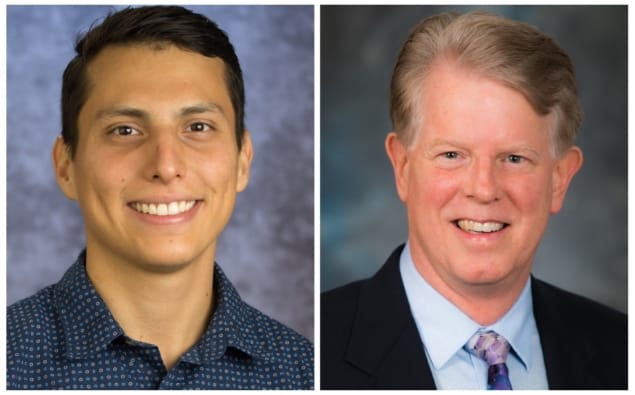
“As the S-values are simply the radiation dose per radionuclide decay, multiplying them by the number of decays gives us the tumour dose,” explains Wesley Bolch at the University of Florida, who led the study. “This database of S-values allows the complexities of tumour dosimetry to be simplified down to the product of two numbers!”
The team also plan to incorporate the results into a community-developed project called MIRDcalc – a software program that will calculate organ doses in nuclear medicine and X-ray CT in a single package.