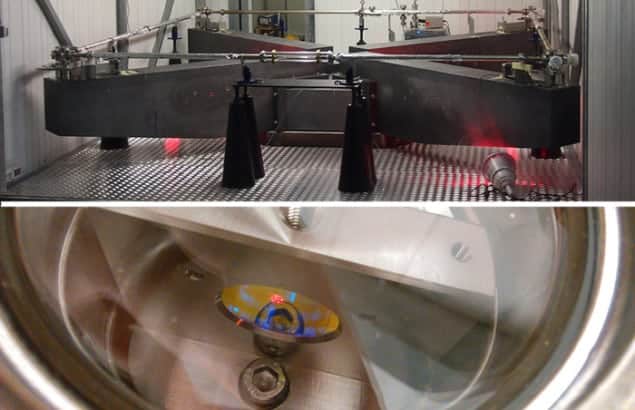
Physicists have started work on a new experiment that aims to measure a very subtle prediction of Einstein’s general theory of relativity known as the Lense–Thirring effect. Describing the distortion of space–time by a spinning mass, the effect has previously been measured by NASA’s LAGEOS satellites to an accuracy of 10%. Now, the Gyroscopes in General Relativity (GINGER) experiment will try to measure the effect under Italy’s Gran Sasso mountain to an accuracy of about 1%.
Einstein’s general theory of relativity says that the motion of a body placed in the gravitational field of another large, spinning object, such as the Earth, will be modified in two ways. One, known as geodetic precession, is due to the curvature of space–time around the Earth. The Lense–Thirring effect, on the other hand, involves the rotating Earth twisting space–time around itself as it turns. First predicted in 1918, the effect is also known as “frame dragging”.
Angela Di Virgilio of Italy’s National Institute of Nuclear Physics in Pisa and colleagues plan to use ring-laser gyroscopes to measure the effect at slightly below the Earth’s surface. These devices use highly reflective mirrors to direct a pair of laser beams in opposite directions around a fixed and very stable loop. The ring works as a resonating cavity for both beams, but each resonates at a slightly different frequency. The beam that tends to travel with the Earth as it rotates takes slightly longer to complete its journey than does the opposing beam. This means that it travels for slightly longer through the resonant cavity. Because the beams have a spread of frequencies, the cavity-length difference translates into two slightly different selected frequencies, and hence a series of beats, which reveal the Earth’s rotation rate.
Tiny modulation
Frame dragging appears as a tiny difference between the rotation rate of the Earth as measured by GINGER and the rotation rate of the Earth as measured by an observer who is not on Earth and in a frame that is fixed to the stars. The latter can be determined very accurately using very long baseline interferometers. What makes the measurement challenging is just how tiny the difference is. The Earth rotates through about 10–5 radians each second but the Lense–Thirring effect leads to a change of only 10–14 radians per second. In other words, the researchers would be looking for variations of one part in a billion.
To achieve this sensitivity, and to track the Earth’s rotation in three dimensions to distinguish between the frame-dragging and geodetic precession, the GINGER design calls for at least three ring lasers, each consisting of a square loop with sides 6 m long, mounted at right angles to one another. Its location in the Gran Sasso National Laboratory, some 1400 m under the Earth’s surface, is also crucial, because it would help to isolate the experiment from wind, rain and other environmental “noise”. Indeed, The world’s best ring-laser gyroscope currently operating in Wettzell, Germany, falls short of the required 10–9 sensitivity by about a factor of 10, as a result of local movements in the Earth’s crust.
To find out whether Gran Sasso provides the necessary isolation, Di Virgilio and colleagues have set up a test apparatus called GINGERino. Installed in the innermost part of the lab, it consists of a square ring laser with 3.6 m-long sides that is mounted on a block of granite secured to the underlying bedrock via a concrete slab. The experiment has so far provided two sets of data, taken in spring and autumn last year, which the researchers say give a “good indication that the underground environment is good and stable”.
Damaged mirrors
However, Di Virgilio says that “there is room for improvement”, and that GINGER still faces significant technical challenges. Crucial to ring-laser experiments is being able to take data over extended periods of time to reduce error. But unwanted noise, partly due to damaged mirrors, has so far limited this “integration time” to just a few tens of seconds. The researchers aim to boost this to around an hour by upgrading the mirrors. They also want to install air-tight doors to limit variations in air pressure within the tunnel housing the experiment. Ultimately, Di Virgilio says that if GINGER is built at Gran Sasso, they will need further funding of €5m, so it can start taking data by around 2019.
GINGER will have to compete against the Laser Relativity Satellite (LARES) – an ongoing mission of the Italian Space Agency – which is aiming at an uncertainty of a few per cent. Di Virgilio points out that LARES, like LAGEOS, requires a separate space mission to map how Earth’s gravitational field varies in space, whereas GINGER does not need such a map.
Heiner Igel, a geophysicist at the University of Munich, says that the biggest challenge facing GINGER physicists is stabilizing the ring’s geometry. “Being under a mountain certainly helps, but the stabilization will still be necessary,” he says.
However, Clifford Will, an astrophysicist at the University of Florida, doubts that GINGER could observe the Lense–Thirring effect, no matter how stable the ring is. He argues that it will be very hard to know the ring’s shape, latitude and orientation all to one part in a billion. “This kind of absolute metrology is very hard to achieve,” he says. “So while there may be compelling geophysical reasons for developing such an instrument, I’m very sceptical that any test of general relativity can be done”.
The GINGERino results have been uploaded to the arXiv server.