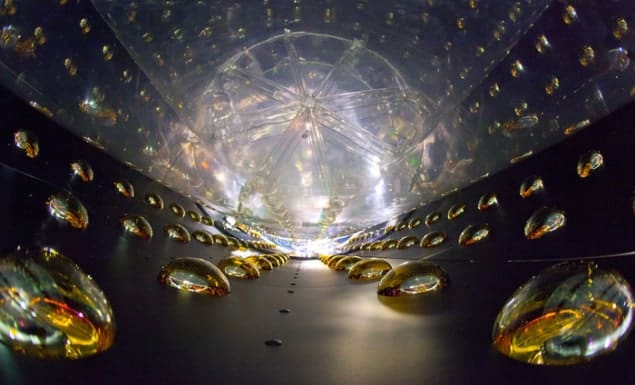
Analysis of more than 300,000 electron antineutrinos emitted by nuclear reactors in China provides the best evidence yet that the flux and energy distribution of such particles do not agree with theoretical predictions. While the disparities could be caused by deficiencies in current models describing neutrino production and detection, a hitherto unknown fourth neutrino could also explain some of the disagreement with theory.
The data were obtained from the international Daya Bay Reactor Neutrino Experiment, which consists of eight antineutrino detectors that look for antineutrinos emitted by six nearby nuclear reactors. This latest measurement was done using six of these detectors, each containing 20 tonnes of a gadolinium-doped liquid scintillator that emits a tiny flash of light when an electron antineutrino interacts with a gadolinium nucleus.
Mysterious bump
Data were collected over 217 days, allowing the team to measure the energies of the antineutrinos to within 1% uncertainty – which the researchers claim is the most precise measurement to date. But instead of agreeing with current models of antineutrino production, the energy spectrum contained a large excess of antineutrinos at an energy of 4–6 MeV with a statistical significance of 4σ.
Although this is less than the 5σ normally required for a “discovery” in particle physics, the existence of this bump is backed up by two other reactor neutrino experiments – Double Chooz in France and RENO in Korea. Both have already seen excesses at 4–6 MeV, with significances of 3σ and 3.5σ, respectively.
Despite the excess at 4–6 MeV, however, the total number of antineutrinos detected at Daya Bay with energies in the 1–7 MeV range was 6% less than predicted by theory. This deficiency was first identified in 2011 by Thierry Lasserre and colleagues at CEA Saclay in France, who evaluated data from a number of different reactor experiments.
According to Lasserre and colleagues, one explanation for the overall deficit of antineutrinos is that the missing particles have oscillated into a hypothetical fourth type of neutrino as they travel from reactor to detector. One candidate is the “sterile” neutrino, which is predicted by certain extensions of the Standard Model.
If they exist, sterile neutrinos would interact extremely weakly, if at all, with ordinary matter, and so would be even harder to detect than conventional neutrinos. However, the existence of sterile neutrinos could be inferred from discrepancies between measured and predicted neutrino fluxes.
More evidence needed
According to Lasserre, who is not part of the Daya Bay collaboration, physicists seeking sterile neutrinos will have to wait several years for better evidence. “We need new experiments dedicated to search for sterile neutrinos, and several of them are currently being realized,” he says. “We may expect new results within the next three years.”
As for the excess of antineutrinos at 4–6 MeV, Lasserre says this anomaly is relatively new, having first been identified in 2014. “Daya Bay now provides the most precise data, and this is a great result, but we don’t have yet any solid explanation of what it means exactly,” he explains.
However, Lasserre adds it is unlikely that the bump is related to sterile neutrinos, but could instead be related to limitations in our understanding of how antineutrinos are produced in reactors or of how the detectors work. Daya Bay Collaboration co-spokesperson Kam-Biu Luk of the University of California at Berkeley concurs. “This unexpected disagreement between our observation and predictions strongly suggested that the current calculations would need some refinement,” he says.
The Daya Bay measurement also provides important information for physicists studying how neutrinos from nuclear reactors oscillate between flavours as they travel long distances to remote detectors. According to the collaboration, such experiments may “need to revisit the models underlying their calculations”. This includes the JUNO detector, which is currently being built 200 km from Daya Bay.
The research is reported in Physical Review Letters.