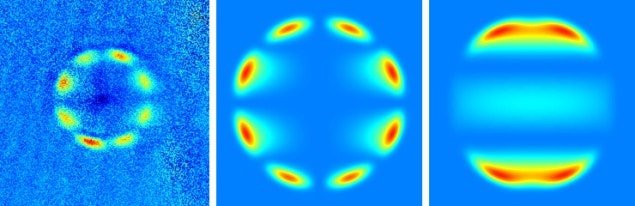
A new study of how light causes diatomic molecules to break apart has revealed significant flaws in the traditional theory describing the photodissociation process. The work has been carried out by physicists and chemists in the US and Poland, and suggests that the dissociation of molecules prepared in pure quantum states is best described by a recently developed quantum-chemistry model. As well as providing further insights into the quantum nature of molecules, the experimental technique could form the basis of a new source of entangled atoms for matter-wave experiments.
Photodissociation occurs when a molecule is blown apart by absorption of a photon, and it has long been used to study the physics and chemistry of molecules. The process usually involves the electric-dipole moment of the molecule coupling to the oscillating electromagnetic field of the photon – although symmetry considerations forbid this interaction in some situations.
The process is usually studied by creating an ultracold, supersonic molecular beam that is irradiated with light from a pulsed dye laser. However, the minimum achievable temperature of such a molecular beam is too high to allow molecular ensembles to be prepared in pure quantum states before dissociation. Instead, what is observed is the average of the dissociation patterns of multiple quantum states. These observations are described very well by the quasi-classical model for electric-dipole dissociation that was developed in the 1960s by Richard Zare and Dudley Hershbach of the University of California, Berkeley, in 1963. Hershbach shared the 1986 Nobel Prize for Chemistry for his work on molecular beams.
Optical lattice
Now, physicist Tanya Zelevinsky of Columbia University and colleagues have done a much more subtle experiment. They confined ultracold (5 μK) strontium-88 atoms in an optical lattice, before bringing them together by photon absorption to produce excited Sr2 molecules. These then decayed rapidly to their lowest-energy (ground) quantum state. These molecules could then be further excited to specific higher-energy bound states, which could then be studied.
Having prepared samples of these molecules in the desired quantum states, the researchers exposed them to pulses of linearly polarized 689 nm laser light. This causes the molecules to break apart and the team measured the trajectories of the ejected atoms. The researchers were also able to prepare pure samples of molecules that cannot dissociate through electric-dipole interactions. As a result, they were able to study weaker, previously unobserved magnetic-dipole and electric quadrupole dissociation processes.
To understand their results, Zelevinsky and colleagues joined forces with quantum chemists at the University of Warsaw, who calculated the expected emission patterns using the quasi-classical approximation.
Complete disagreement
“They basically all disagree,” says Zelevinsky. “In some cases, it wasn’t surprising from what people have suggested in the past, but, in some cases, we were a bit surprised that [the quasi-classical approximation is] not applicable.” Zelevinsky points out that the angular distributions of the dissociation patterns often change as the energy of the photons increases, and that many of the distributions are not cylindrically symmetric about the polarization axis of the laser pulse. “All of that is to do with us creating two different final end states that undergo matter-wave interference,” she explains.
The Warsaw researchers also predicted the emission patterns using a more sophisticated, fully quantum model that they co-developed in 2012, and found much better agreement (see figure). This new model could even predict the fragment distribution for the forbidden transitions correctly.
The team now plans to look at molecules in higher-energy states to study how the quantum model becomes obscured by more classical behaviour. “In all of the energies we’ve done, it’s very distinctly quantum,” says Zelevinsky.
Matter waves
The atomic fragments emerge from the dissociation process in an entangled state, and Zelevinsky suggests that the experimental technique could provide a useful source of matter waves for atom optics experiments. The method could also be used to determine the binding energies of molecules in specific states.
“It’s beautiful work,” says experimental atomic physicist Simon Cornish of Durham University, who was not involved in the study. “The quality and the clarity of the results are just outstanding.”
The research is described in Nature.