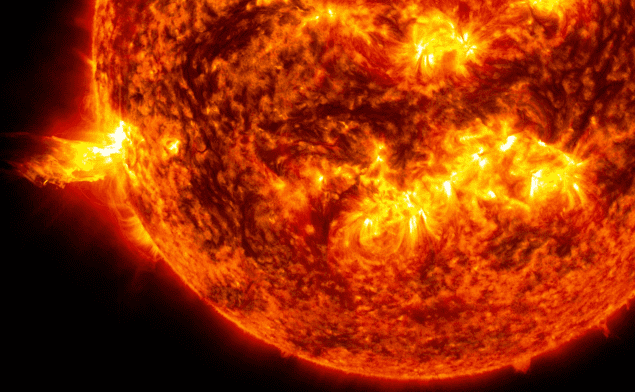
Neutrino fluxes generated by nuclear reactions inside the Sun have been measured more precisely than ever before. Using a detector housed under the Gran Sasso mountain in central Italy, the Borexino collaboration has captured neutrinos from four different reactions involved in the creation of helium-4 from hydrogen. The results confirm the nuclear origin of solar power and could help to pin down the abundance of elements heavier than helium inside the Sun.
Some 99% of the Sun’s energy is generated through reactions that begin with the fusion of two protons. This “proton-proton chain” proceeds via a number of different routes. The most common route involves the fusion of two helium-3 nuclei, while others feature the temporary production of beryllium-7 and one the creation of boron-8. Occasionally, the chain can also start with the fusion of two protons and an electron (pep).
To detect the neutrinos created in each of these reactions, Borexino employs a 300 ton sphere of hydrocarbon liquid scintillator. Any neutrino scattering off an electron inside the scintillator generates a flash of light that is picked up by an array of photomultiplier tubes. Crucially, the experiment has extremely low levels of radioactive interference, thanks both to the 1400 m of rock above the Gran Sasso National Laboratory – which blocks incoming cosmic rays – and to the ultra-pure materials used to contain and shield the detector.
Lowest uncertainties
Using data collected between 2012-16, the international collaboration reports values for the fluxes of neutrinos generated from: the initial fusion of two protons; the capture of an electron by beryllium-7; the beta decay of boron-8; and pep fusion. As pointed out by collaboration spokesman Marco Pallavicini of the University of Genoa, Borexino has already measured each of these quantities before, and indeed the boron-8 flux has been pinned down more precisely by detectors employing large tanks of water – the Sudbury Neutrino Observatory (SNO) in Canada and Super-Kamiokande in Japan. The novelty, he says, is to have measured all four quantities with the same set of data and to have done so with the lowest uncertainties yet for the proton-proton, beryllium-7 and pep reactions – 9.5%, 2.7% and 15% respectively.
It is an impressive suite of measurements
Gabriel Orebi Gann
Gabriel Orebi Gann of the University of California, Berkeley, who works on SNO and other neutrino experiments, praises the research. She says that the latest results underline Borexino’s reputation for furthering our understanding of both the Sun and neutrinos themselves. “It is an impressive suite of measurements,” she says.
Metallicity puzzle
Aldo Serenelli of the Institute of Space Sciences in Barcelona points out that the new results yield a solar luminosity – derived from the total power generated by nuclear reactions in the Sun – that is within 10% of the measured value. He also says that the new data “contribute marginally” to solving the “metallicity puzzle” – in other words, establishing just how abundant heavier elements such as carbon, nitrogen and oxygen are in the Sun. The Borexino results, he explains, point to a slightly higher core temperature that would tend to favour relatively high abundances but cautions that the higher temperature can be explained in other ways.
The new data are also relevant to fundamental physics. The collaboration has determined the survival probability of solar electron neutrinos at different energies, thereby, it says, “probing simultaneously and with high precision the neutrino flavour conversion paradigm, both in vacuum and in matter-dominated regimes”.
In future, Serenelli argues there is a chance that Borexino could measure neutrinos from the carbon–nitrogen–oxygen (CNO) cycle. An alternative to the proton-proton chain that uses carbon and nitrogen nuclei to catalyse the conversion of hydrogen into helium, CNO is thought to provide most of the energy for more massive stars. And although it would only generate about 1% of the Sun’s output, observation of the associated neutrinos should settle the metallicity puzzle, says Serenelli, because the flux would be very sensitive to the presence of heavier elements in the solar atmosphere.
Thermal coat
Borexino’s chance of bagging such neutrinos was boosted in 2016 after the collaboration surrounded the detector with a thermal coat. Previously, temperature changes within the lab had set up convection currents in the scintillator that would transport radioactive impurities from the nylon vessel surrounding the liquid into the core of the detector. But now, according to Serenelli, the collaboration is in a better position to monitor the relevant background (from bismuth-210) and so potentially measure CNO neutrinos. “There are high expectations they will succeed,” he says.
To maximize the odds of success, Pallavicini says that he and his colleagues would ideally like to further raise the purity of the liquid scintillator. But that option is complicated by the fact that the experiment may have to shut down for good by 2020 to comply with an environmental law concerning groundwater.
However, Borexino is not the only experiment potentially capable of detecting CNO neutrinos. One is SNO+, a revamped version of SNO – due to start taking data in 3-4 months’ time – that will contain liquid scintillator rather than heavy water and which Orebi Gann notes will be larger and deeper than Borexino. “The primary goal of SNO+ is a search for neutrinoless double beta decay,” she says. “But if radioactive backgrounds are low enough then SNO+ could have sensitivity to the CNO cycle”.
The research is described in Nature.