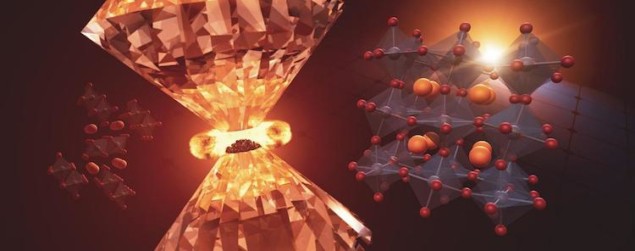
Solar cells made from lead halide perovskites are good at converting solar power into electricity and relatively straightforward to manufacture. Unfortunately, they’re also unstable at room temperature and ambient humidity, which is something of a drawback for devices that tend to be located outside. Now, however, researchers at the US Department of Energy’s SLAC National Accelerator Laboratory and Stanford University may have found a solution. Their new technique involves pre-treating the material at high pressures and temperatures, and its developers say it could be scaled up for industrial production.
Perovskites are crystalline materials with an ABX3 structure, where A is caesium, methylammonium (MA) or formamidinium (FA); B is lead or tin; and X is chlorine, bromine or iodine. They are promising candidates for thin-film solar cells because they can absorb light over a broad range of solar spectrum wavelengths thanks to their tuneable bandgaps. Charge carriers (electrons and holes) can also diffuse through them quickly and over long distances. These excellent properties give perovskite solar cells a power conversion efficiency (PCE) of more than 18%, placing them on a par with established solar-cell materials such as silicon, gallium arsenide and cadmium telluride.
One of the most efficient perovskites for solar cell applications is composed of caesium, lead and iodine. This material, CsPbI3, has four possible phases: a yellow room-temperature non-perovskite phase (δ), plus three black high-temperature perovskite-related phases in which the crystal takes on a cubic (α), tetragonal (β) or orthorhombic (γ) structure. While the black phases are efficient at converting sunlight into electricity, heat and humidity quickly make them revert to the yellow phase, which is useless for photovoltaic applications.
High pressure squeeze
The SLAC-Stanford researchers have now shown that it is possible to nudge this yellow phase into an efficient and stable black configuration. Led by Yu Lin, Wendy Mao, Hemamala Karunadasa and Feng Ke, they did this by placing crystals of the yellow phase between the tips of a diamond anvil cell (DAC) and subjecting it to pressures of 0.1 to 0.6 GPa at a temperature of up to 450 °C. They then rapidly cooled the material down and removed the sample from the DAC.
Synchrotron X-ray diffraction and Raman spectroscopy measurements showed that this treatment yielded a version of orthorhombic γ-CsPbI3 that was stable in the presence of moisture (at a relative humidity of 20-30%) and remained efficient at room temperature for 10 to 30 days. This is a significant improvement over earlier efforts to stabilize the bulk black phase at room temperature using, for example, applied strain, surface treatments and changes to the material’s chemical composition – all of which produced good results only when the environment remained moisture-free.
“This is the first time that pressure has been used to control this material’s stability [under ambient conditions],” Lin says. “Now that we’ve found this optimal way to prepare the material, there’s potential for scaling it up for industrial production, and for using this same approach to manipulate other perovskite phases.”
Stabilizing the γ-CsPbI3 phase
Theoretical studies show that the secret to the SLAC-Stanford team’s success lies in the way the high-pressure, high-temperature treatment affects distortion within the perovskite lattice. Other researchers had previously identified three types of distortion: distortions of the BX6 octahedral units making up the material’s crystalline structure; B-cation displacements within the octahedra; and a tilting of individual BX6 octahedra relative to one another to create rigid corner-linked units. Of these three types, the third, known as octahedral tilting, is the most common.

Durable perovskite solar cells
Two years ago, researchers led by Henry Snaith at the University of Oxford in the UK calculated that the octahedral tilt of the [PbI6]4− cation dramatically affects the formation energies of the three α, β, and γ perovskite phases of CsPbI3. Among these perovskite phases, the γ phase has the largest octahedral tilt, and thus the lowest energy.
In the current study, Chunjing Jia and Thomas Devereaux of the Stanford Institute for Materials and Energy Sciences (SIMES) used first-principles density functional theory calculations to show that applying pressure to the perovskite affects its tilt. More specifically, the pressure treatment makes it possible to control the relative energy difference between the desired γ-CsPbI3 phase and a competing non-perovskite phase (δ- CsPbI3) – and, ultimately, to stabilize the former.
The work is detailed in Nature Communications.